Robert Langer, ScD, is the David H. Koch Institute professor at MIT and a co-founder of Moderna, the pharmaceutical company behind a COVID-19 mRNA vaccine. In this Q&A, he tells us about the present and future of these versatile vaccines.
GEN: The technology behind mRNA vaccines has been available for decades, but it was only with the pandemic that we first saw a publicly available mRNA vaccine. Why didn’t this happen sooner?
Langer: While it is true that over the last thirty years hundreds of scientists have worked on developing mRNA vaccines and therapeutics, real breakthroughs in making an effective and commercially viable mRNA vaccine were greatly accelerated by the COVID-19 pandemic. It’s important to realize that Moderna and others like BioNtech and Curevac were in clinical trials for multiple different vaccines and therapeutics at the time the COVID-19 crisis started in late 2019/early 2020.
In fact, Moderna had eight vaccines (including a personalized cancer vaccine, a vaccine against Zika virus, and a dual vaccine against metapneumovirus and a type of parainfluenza) in human clinical trials at that point. Moderna recognized the incredible and unique opportunity to quickly meet the global demand for a COVID-19 vaccine by leveraging our mRNA tools and technologies. All the necessary pieces were in place, such as the correct mRNA chemistry and the development of nanoparticles to protect the messenger RNA (this actually had part of its origins in our MIT lab for which I received the Millennium Technology Prize in 2008). So the organization refocused efforts on bringing a COVID-19 mRNA vaccine to patients as quickly as possible without compromising public safety.
Moderna scientists identified the ideal protein candidate on the coronavirus SARS-CoV-2 virus (the spike protein), determined the mRNA sequence necessary to encode for this protein and, six months later, was given FDA clearance to proceed.
The many advantages of mRNA versus traditional vaccines prompted scientists to use mRNA vaccines to fight SARS-CoV-2. These advantages include the ability to quickly update the vaccine as new variants emerge, the ability to develop combination vaccines to fight several variants (and pathogens) simultaneously, and the ability to rapidly scale to serve a global population.
What’s more, Moderna’s mRNA platform generates antigens with superior biological fidelity and a greater success rate than traditional vaccines—all in record time. mRNA vaccines don’t require a giant manufacturing plant to produce them. All mRNA vaccines and therapeutics can be produced at the same location via the same process.
GEN: What are some other diseases or disorders against which mRNA vaccines can provide protection?
Langer: Moderna mRNA vaccines are already in the works to reduce the health risks of latent viruses like Epstein-Barr virus (EBV) and cytomegalovirus (CMV) and to tackle additional areas of unmet need, including an all-in-one mRNA vaccine to treat COVID-19, seasonal flu, and respiratory syncytial virus (RSV). Additionally, Moderna plans to develop mRNA vaccines to help patients beat Herpes simplex virus (HSV), MS, cancer, and HIV once and for all.
HSV, CMV, and EBV are large viruses that gain entry into cells via multiple proteins. Identifying which proteins to target remains a challenge, as does the development of adjuvants to fine-tune the body’s immune response. In the case of HIV, the situation is particularly complex.
Simply put, the immune system must be rigorously trained to produce a specific type of antibody (broadly neutralizing antibody or bnAbs), that has been found to be effective at fighting HIV. Further, a truly protective HIV vaccine will likely require a combination of antigens to stimulate the formation of multiple bnAbs classes.
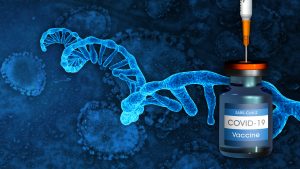
Therefore, mRNA vaccines are limited only by the immune system’s own ability to fight the pathogen. Once an ideal protein candidate is identified, it is a relatively simple process to identify the mRNA needed to encode for those proteins.
GEN: Can mRNA vaccines provide universal protection against a family of viruses, such as coronaviruses?
Langer: Theoretically, yes. AI, machine learning, and robotic process automation (RPA) are technologies that can help us more rapidly catalog common familial viral elements, predict potential variations and mutations, and identify antigen-protein targets—all while eliminating human error.
Further, these technologies can accelerate the process of identifying the ideal, most productive mRNA sequence needed to generate those proteins. Over time, AI/machine learning refinements will continuously improve the range and effectiveness of this approach by providing ever-larger datasets to draw from.
GEN: Do mRNA vaccines have the potential to combat antimicrobial/antibiotic resistance?
Langer: Yes. Antimicrobial and antibiotic resistance arises as a natural evolutionary process, the result of genetic variation (“errors”) and mutation in the pathogen, giving rise to novel traits. Organisms that develop traits which make them drug-resistant naturally survive and thrive.
First, mRNA vaccines have the potential to help combat antimicrobial and antibiotic resistance by reducing our reliance on traditional antibiotics. Additionally, Moderna’s mRNA platform helps combat resistance by identifying the most desirable and persistent antigens for targeting, by improving the prediction of new strains, and by accelerating the production of vaccines to defeat those strains.
Finally, by training the immune system to look for specific surface proteins on the bacterium, an mRNA vaccine more effectively targets specific pathogens while avoiding the issue of damaging a patient’s “good” bacteria with increasingly toxic antibiotics. For instance, through the selection of a “coat protein” as the target-antigen, an mRNA vaccine against a drug-resistant strain of malaria has already yielded encouraging results.
GEN: On the whole, mRNA vaccines are safer, more efficient, and easier to produce than “traditional” vaccines. However, like with all technologies, there is always room to improve its accessibility, affordability, efficacy, and safety. Cold storage requirements and side effects including possible allergic reactions are some of the issues impacting uptake of these vaccines. Are there any solutions to these (or any other) issues that researchers are currently working upon?
Langer: Moderna is leveraging AI and machine learning technologies to identify ways to make mRNA vaccines safer and more durable (with simpler refrigeration requirements) by minimizing the length of mRNA strands. Moderna is implementing a multi-pronged approach to ease the substantial global disease burden by making mRNA vaccines more widely accessible, affordable, efficient, and safe.
Moderna’s mRNA Access program aims to accelerate the creation of new mRNA vaccines through collaboration with global partners. mRNA Access lets outside researchers leverage Moderna’s platform to develop mRNA vaccines against emerging and neglected infectious diseases around the world.
This strategy exponentially expands our brain trust, making the discovery of safer and more efficient vaccines an inevitable outcome. To help ensure the success of our mission to combat the top respiratory diseases worldwide, Moderna has entered into ten-year supply agreements with strategic countries.
Finally, Moderna is expanding its COVID-19 vaccine technology patent-free into 92 additional low- and middle-income countries as well as building an mRNA manufacturing facility in Kenya.
In addition, at MIT, we are working on ways of creating self-boosting vaccines that can be given in a single injection that does not require the patient to return for boosters. We are also developing ways to create more stable nanoparticles, as well as microneedle patches that could be shelf stable and shipped around the world.
GEN: Recently, there has been discussion about developing vaccines that can be administered as a pill or a nasal spray. Is this method of delivery possible for mRNA vaccines?
Langer: The possibilities for vaccine administration are endless so long as an appropriate, effective delivery mechanism can be found that protects the mRNA from the local environments (e.g., mucus, saliva, stomach acid [HCL]) yet facilitates the delivery of mRNA into the cell. While the gold standard for vaccine administration is through an intramuscular shot in the arm, intranasal COVID-19 vaccines have already been shown to elicit strong cellular immune response in humans.
Additionally, at MIT, Gio Traverso and myself recently developed a self-orienting mRNA “milli-injector” capsule that uses a polymer coating to deliver mRNA to the stomach via milli-needle injections in the gastric lining, thereby avoiding damaging acids. The experiments successfully produced proteins in gastric mucosa, as well as systemic uptake though, due to volume restrictions, their effectiveness may be limited to diseases of the gastrointestinal tract.
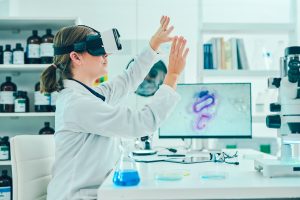
A nasal vaccine’s formulation and delivery method must be precisely tuned and would require amplification via immunostimulants. Despite this, several vaccine companies are experimenting with intranasal delivery by tweaking nasal mucosal toxicity, mucoadhesion, and pH. AstraZeneca and the Chalmers University of Technology have initiated mRNA-based nasal spray vaccine development against SARS-CoV-2, where nasal vaccines make the most sense since viral invasion happens via the nasal mucosa.
Advantages of a pill or nasal spray include reduced patient hesitancy and increased compliance. Specific advantages of an intranasal spray include ease of combining vaccines, simple vaccine swapping to accommodate variants, a combined mucosal and systemic immune response, protection at distant mucosal sites, and a faster onset of a strong immune response.
GEN: What are some other ways that mRNA vaccine technology can improve human wellbeing that you believe hasn’t yet had enough attention or curiosity?
Langer: An mRNA vaccine enables the patient to produce proteins, but these proteins don’t have to be used to stimulate the immune system to fight disease. mRNA vaccine technology can be used to replace missing proteins in patients suffering from inherited metabolic disorders (of which hundreds exist, such as phenylketonuria). These potentially debilitating diseases are caused by an enzyme deficiency; the body is unable to manufacture or break down certain proteins.
Phenylketonuria (PKU) results in low levels of the enzyme phenylalanine hydroxylase, a protein that breaks down phenylalanine. Without it, phenylalanine builds up in the body, often to toxic levels, even causing brain damage. Current treatment is simply the dietary reduction of foods that contain phenylalanine (which includes much more than you think: all meats, milk, eggs, cheese, nuts, soy, beans, and more). The dietary requirements are so severe as to require supplementing with a special drink to ensure the essential nutrients.
Similarly, an mRNA vaccine may be used to potentially help treat depression by enabling patients to boost their levels of serotonin or dopamine to elevate their mood or reduce anxiety. In short, an mRNA vaccine can be used to express any protein and possibly treat almost any disease.
GEN: What advice would you give to budding researchers interested in studying and working on mRNA vaccines?
Langer: I think it’s important to realize that there are few limits. Whether it’s mRNA vaccines or anything else, I always tell my students to dream big dreams, dreams that can change the world. But if you do that you may run into obstacles. When I first discovered a way to deliver macromolecules including nucleic acids from small particles, I was ridiculed by the scientific community. My first nine research grants were rejected and no engineering department in the world would hire me for a faculty position. But I didn’t give up and I always tell my students to never give up either.
GEN wishes to thank David Olajide, consultant at CURZONPR in London, for providing the raw material for this article.