Organoids—self-organizing, self-reproducing structures that serve as 3D in vitro models—date back to 2009. Back then, researchers at the Hubrecht Institute led by Hans Clevers, PhD, introduced a method for culturing miniature guts from single mouse intestine stem cells. Now, thanks to additional research by Clevers and many other scientists, the basics of organoid culturing systems have become well established. In these systems, stem cells are exposed to growth factors and supported by a 3D matrix, typically a hydrogel of some kind, giving rise to functional miniature organs.
Today, organoids that behave like various kinds of organs are routinely generated from human stem cells. Organoids are considered superior to immortalized cell lines in recapitulating the 3D architecture, heterogeneity, and functionality of primary tissues, and hence they are thought to be more physiologically relevant for modeling human diseases and predicting therapeutic responses.
Opportunities for humanized in vitro models are expanding thanks to recent legislation. In late 2022, President Biden signed the FDA Modernization Act 2.0 into law. It authorizes the use of certain alternatives to animal testing, including cell-based assays and computer models, to investigate the safety and effectiveness of a drug prior to clinical trials. It also provides more resources to the FDA to drive collaboration to advance the standardization of organoids, organ-on-a-chip devices, and other 3D models.
Addressing the gaps
“To advance the use of organoids, you need an ecosystem of solutions that work together,” maintains Shantanu Dhamija, vice president for strategy and innovation at Molecular Devices. “Partnerships that enable complete solutions versus piecemeal offerings that customers need to assemble will address a number of tactical issues.”
To facilitate 3D biology applications, Molecular Devices offers the ImageXpress Confocal HT.ai High-Content Imaging System. It is an automated microscope for high-content screening. With its high excitation power, the microscope permits short exposure times and facilitates faster imaging. Supporting analysis software, IN Carta, uses parallel processing to analyze 3D images 40 times faster than a normal analytical workflow.
Dhamija points out that workflows for generating, monitoring, and using organoids generally fall into two categories, based on throughput. The first category includes workflows for fully automated, high-throughput organoid generation and assay management. In these workflows, scheduling software ties together a string of imagers, liquid handlers, centrifuges, and other instruments to automate critical steps such as dispensing Matrigel into domes, passaging, or dosing.
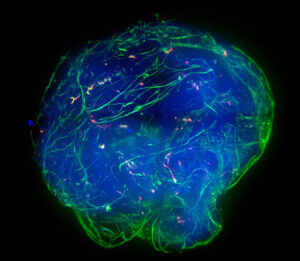
The second category, Dhamija continues, includes workflows for lower throughput operations. These workflows might seem less desirable, but there are situations in which they might be preferred. “The BioAssemblyBot 400 (BAB400), an innovative 3D bioprinter with a six-axis robotic arm, is a self-contained solution that offers lower throughput, but in a more compact form,” Dhamija explains. “By adding a high-content imager and an incubator, the six-axis arm with removable tools allows the system to become a self-contained work cell for organoid picking, dispensing, monitoring, and incubation.”
Growing organoids at the scale needed for screening and drug discovery remains challenging. This need prompted Molecular Devices to acquire U.K.-based Cellesce and its proprietary bioreactor technology for scaling up organoid production for 10,000–50,000 compound runs. Customers specify the types of organoids required, provide an organoid line, or send patient tissue for expansion.
“This is a game changer,” Dhamija declares. “A lot of interest exists in using organoids in drug discovery. But usage is still only about 20%, and screens have not been large.” Molecular Devices is also working with HUB Organoids, a not-for-profit organization founded by the Hubrecht Institute, to validate next-generation instruments for automated intestinal organoid screening.
“We want to industrialize organoids to enable scale, reproducibility, low cost, and low error bars,” Dhamija relates. “Before organoids can move into applications such as precision medicine, we need to conquer screening. As new therapeutic modalities become more human-like, the less applicable or insightful animal models become.” According to Dhamija, another way Molecular Devices is demonstrating its “fundamental belief in organoids” is by collaborating with Vienna-based HeartBeat.bio to automate the generation of cardiac organoids (cardioids).
Utilizing microfluidics
Mimetas takes comprehensive in vitro tissue modeling to another level. “Looking in a more inclusive manner at disease offers the advantage of taking into account the crucial juxtacrine interplay between different cell types,” explains Paul Vulto, PhD, CEO of Mimetas. “For example, you can study aspects like immune cell recruitment, which is crucial to supporting novel immuno-oncology therapies, and find novel targets for immune disorders and fibrosis.”
To support this approach to in vitro tissue modeling, Mimetas offers the OrganoPlate, a microfluidic technology. In vitro tissue models typically comprise epithelial tubules, stroma, vasculature, and immune cells supported by a 3D extracellular matrix gel and are under continuous perfusion. The OrganoPlate generally contains 64 microfluidic chips and can be fully automated.
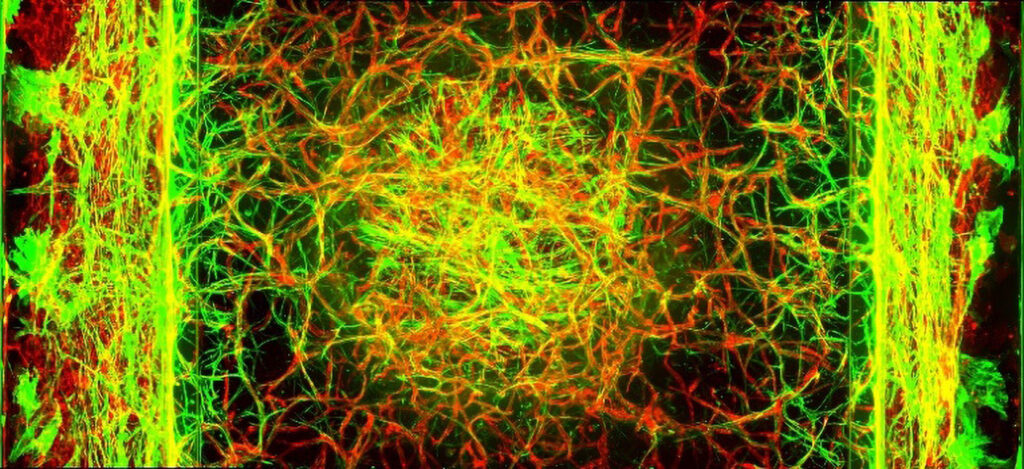
“Due to our patented PhaseGuide technology, we can recreate tissue physiology without artificial membranes,” Vulto asserts. “Our models are being used for understanding disease biology, new target and compound discovery, prioritization and selection, as well as ADME/Tox preclinical studies.” Models are available for the intestinal tract, liver, kidney, lung, central nervous system, and other tissues. The company uses them to study inflammatory and fibrotic diseases as well as cancer.
A number of models are available in OrganoReady format, meaning that live tissues are shipped to customers. Products include adult stem cell–derived intestinal organoids and kidney tubules, blood-brain barrier endothelium, Caco2 tubules, and blood vessels. OrganoReady products are used in preclinical development. Typical activities include tests of blood-brain barrier transport, gut absorption, or toxicity.
“We offer throughput and compatibility in a membrane-free format and comprehensive tissue modeling and perfusion flow,” Vulto notes. “We place the disease model central to drug discovery and offer our expertise through our partnership programs, services, and product offerings.”
About 60 scientists work daily on developing new models and deploying them for the development of therapies against today’s unsolved diseases. According to Vulto, the company is making notable progress in hepatocellular carcinoma, liver metastasis, chimeric antigen receptor T-cell therapy assays, infectious diseases, inflammatory bowel disease, chronic kidney disease, and other indications.
Screening services
The Crown Bioscience biobank contains over 200 patient-derived organoid (PDO) models for six oncological indications. Notably, for four indications, patient-matched normal (non-cancer) models are available. In addition, offered irritable bowel disease PDO models can be used in testing anti-inflammatory treatments and regenerative medicine approaches.
Crown Bioscience also used its biobank of patient-derived xenografts biobank to generate patient-derived xenograft organoid (PDXO) models. Around 400 PDXO models are available for 18 indications, and the offering is expanding (PLoS One 2023; 18(1): e0279821). “We have matching in vivo models for the PDXO models for validation or efficacy studies,” says Mariusz Madej, PhD, associate director of organoid screening, Crown Bioscience.
Authentication analyses ensure that genetic drift and contamination are absent, and that historical data from primary tissues are comparable. “We see very high correlations over the passaging of the organoids,” Madej continues. “The PDXO models have preserved the genetic background and gene expression when compared to their parental PDX models, and responses are similar between in vitro and in vivo models during pharmacological profiling.
“But you need to follow the standard procedures so that the organoids do not lose the stem cell population. Culture conditions are extremely complex. Passaging and freezing methods are crucial for maintenance of long-term, high-quality cultures.”
As a contract research organization (CRO), Crown Bioscience provides in vitro, in vivo, and biomarker analysis services. Organoids can be used during early-stage drug discovery (to perform high-throughput screens of thousands of compounds) and also during drug development (to test lead compounds for efficacy, safety, side effects, combinatorial effects, and pharmacokinetics).
With their high drug response predictivity, organoids also allow for “in vitro clinical trials” and downstream biomarker analysis for the identification of responsive and nonresponsive patient populations. Large-panel screening capabilities planned for release later this year will shorten timelines and reduce costs.
The company offers several well-characterized co-culture assays with immune and stromal cells, and it intends to further expand the co-culture complexity. The additional use of CRISPR-Cas technology enables development of knock-down, knock-out, and knock-in models for specific applications. High-content imaging allows both visualization and quantification for a deeper dive into complex morphology and biological changes.
Specialized culture reagents
Organoid culture conditions can be controlled to support innate cellular programming of progenitor cells and drive the organization of in vitro structures, recapitulating structural and functional characteristics of tissues to an unprecedented degree.
According to Jenna Moccia, PhD, director of product management, epithelial biology, Stemcell Technologies, some of the major challenges of organoid adoption go hand in hand with the models’ potential strengths. For example, culture complexity is information rich, but hard to interpret cleanly; patient-to-patient representation means being able to distinguish the effects of different compounds on different segments of the population, but also presents a challenge for standardization.
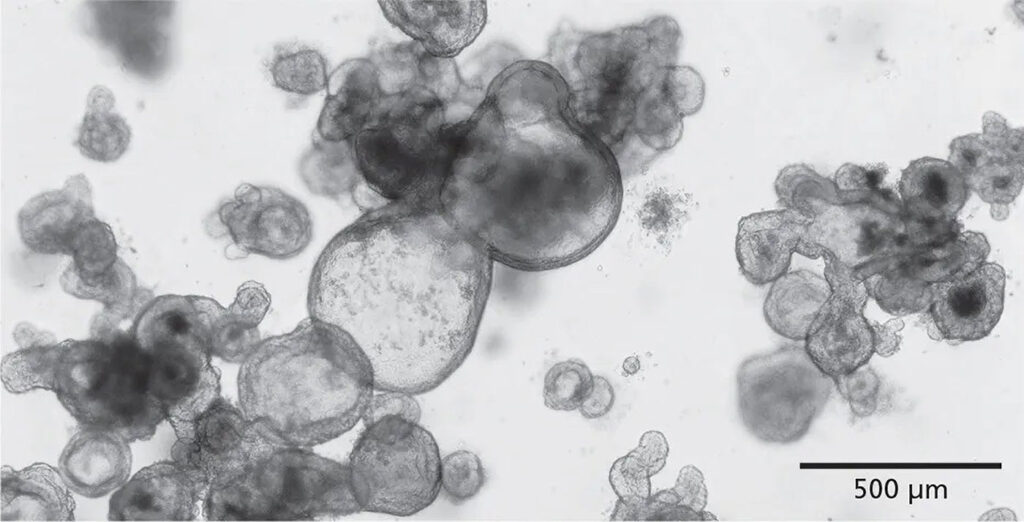
“It will take time and energy to optimally apply organoids to all of the areas where they could be valuable research tools,” Moccia notes.
Stemcell Technologies develops specialized cell culture reagents for both organoids derived through directed differentiation of pluripotent stem cells (PSCs) and organoids derived from culture of adult tissue–resident epithelial stem or progenitor cells. The reagents also support organoids modeling tissues from all three germ layers: the ectoderm, mesoderm, and endoderm.
The company also offers cryopreserved mouse organoids for intestine, liver, and pancreas, and plans to eventually provide a larger variety of PSC-derived organoids. Organoid-based services for evaluating compound toxicity are provided by its in-house CRO, Contract Assay Services.
“Organoids are very versatile culture systems,” Moccia says. “Our work is focused on bringing optimized and standardized reagents to scientists to support new tissue models, and also in tailoring the biological models we already support to applications seeing surges in use, such as drug discovery and diagnostics development.
“Five years ago, intestinal organoids derived from adult tissue were dominated by intestinal progenitors and lacked much of the functionality of the adult intestinal epithelium. Now we have culture conditions that support differentiation of these cultures, and they exhibit mature characteristics such as a typical brush-border. I also anticipate the metabolic functionality of hepatic organoids to progress significantly over the next few years.”
Getting models to gel
Extracellular matrix (ECM) hydrogels, such as Corning Matrigel matrix, are essential for organoids to form in vitro. “ECMs help cells to orient themselves and polarize so that they better recapitulate the in vivo environment,” says Hilary Sherman, a senior applications scientist at Corning Life Sciences. “In fact, Matrigel matrix is such a widely used ECM that we recently added another production line to meet demand.”
Many varieties of Matrigel matrix are available today. For instance, Matrigel Matrix for Organoid Culture contains optimized matrix qualities for organoids. Each lot is tested for stiffness, the ability to form domes, and more to ensure that the product is specifically geared for organoid growth.
When cold, Matrigel matrix is a viscous liquid. As it warms, it becomes a gel that can be used to provide a scaffold or encapsulate cells. A challenge can be temperature control; everything involved in the process must be kept cold. In some cases, that is done manually, or specially designed equipment can be used to automate some steps of the process. For example, the Corning Matribot bioprinter has a temperature-controlled print head to allow for dispensing and printing hydrogels such as the Matrigel matrix.

Another product, the Corning Elplasia 12K flask, has the footprint of a T-75 flask, but the bottom surface contains 12,000 microcavity wells that feature an ultra-low-attachment coating. During seeding, the cells settle into the cavities, and based on the cavity geometry, a single uniform spheroid forms in each cavity.
More products are in the works, such as a completely synthetic hydrogel, Corning Synthegel 3D matrix, to scale up induced PSC spheroids. “Customers are doing all types of different and innovative protocols,” Sherman relates. “We help support their needs by providing as many product variations as possible.”
Organoid work is very challenging because it is nuanced. For example, it can vary from experiment to experiment as well as among different laboratories. As Sherman states, “Developing better protocols or finding ways to standardize existing ones to reduce the biological variability is going to be essential.”