The past decade has seen a dramatic acceleration in DNA sequencing capability, together with significant advances in genome editing tools such as CRISPR. However, DNA synthesis technologies have been slow to catch up.1

The predominant existing chemical method, phosphoramidite synthesis, was first established in the 1980s. It relies on building DNA strands one nucleotide at a time. However, its innate error incorporation rate limits the useful length of synthesis to around 200–300 nucleotides with extensive and time-consuming assembly and post-synthesis quality control. It can also be challenging to synthesize repetitive and GC-rich sequences, due to their propensity to form secondary structures. Enzymatic DNA synthesis methods are also being developed, but the efficiency and the accuracy of these techniques remain open questions, particularly for sequences longer than 300 nucleotides.
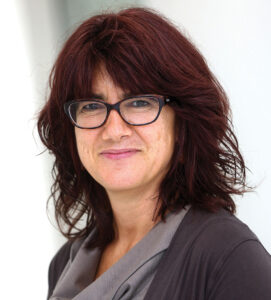
Improved silicon chip technology has the potential to advance a new generation of synthesizers. With this technology, a chip can incorporate up to 10,000 independently controlled thermal pixels, each of which can act as a reaction site for either chemical or enzymatic DNA synthesis. Thermal control of pixels allows the method of synthesis to be updated from existing acid deprotection approaches to new thermally labile protecting groups. It also allows long sequences to be assembled on-chip in a process biased in favor of error removal at every step in the assembly process.
Thermal chip technology promises to increase fidelity by orders of magnitude compared with current technologies by extending the length of synthetic sequences to many thousands of nucleotides. In addition, thermal chip technology could enable synthesizers to uniquely deliver error-free double-stranded DNA, significantly reducing the time taken for post-synthesis processing and purification.

The synthesis and the assembly of longer DNA sequences typically involve specialized equipment and immoderate costs. Consequently, much of this work is outsourced to centralized facilities, taking control out of the hands of scientists. However, this work could be done in-house with sequencers based on thermal chip technology. This technology offers not only highly parallelized synthesis of accurate, long DNA strands, but also a means of creating miniaturized (and affordable) “plug and play” devices.
The coming generation of accessible DNA synthesizers promises to broaden the democratization and transformation of drug discovery and development instigated by desktop PCR thermocyclers and DNA sequencers, with applications across many therapeutic modalities. There are several areas in drug discovery and development where novel synthesis technologies could have immediate and far-reaching impact.
Recombinant monoclonal antibodies
Monoclonal antibodies are an extremely attractive modality for drug development because they have high binding specificity and are relatively easy to manufacture. Since the first monoclonal antibody therapy was approved by the U.S. Food and Drug Administration in 1986, the market for therapeutic antibodies has grown exponentially to more than $100 billion. Today, antibodies represent more than 80% of all biopharmaceuticals, covering a wide range of disease areas including oncology, immunology, and infectious diseases.
Recombinant antibody technology2 has enabled significant improvement in the quality and scope of antibody preparation, leading to the engineering of antibodies and antibody fragments for specificity, reactivity, isotype, and fragment conformation. Parallel synthesis of many thousands of individual synthetic DNA sequences at scale has the potential to further revolutionize antibody discovery and engineering through the generation of rationally designed, codon-optimized antibody libraries with 2- to 10-fold more variants than conventional methods.3
Access to highly accurate synthetic DNA sequences of 3–4 kb on demand will give researchers even greater ability to engineer novel structures and specificities, allowing the rapid generation of highly diverse libraries and efficient optimization cycle times once potential hits have been identified.
Synthetic DNA also expands the possible universe of variable sequences, going beyond what can be found in libraries derived from human or animal donors. This offers new avenues for “drugging the undruggable” through the creation of antibodies against targets that are difficult to bind with conventional small molecules, such as G protein–coupled receptors.
Biomolecular therapeutics
Synthetic DNA technology expands the possibilities for the development of improved or entirely new biomolecular therapies, including enzymes, peptides, RNA catalysts, and DNA and RNA aptamers.
On-demand DNA synthesis could aid drug discovery through the rapid generation of libraries of genetically encoded novel peptides,4 particularly for addressing challenging targets such as protein-protein interactions. Combined with DNA barcoding5—another technology reliant on access to synthetic DNA at scale—this approach also makes hit identification and deconvolution straightforward.
Recent advances in computational biology, such as DeepMind’s AlphaFold, have expanded the capacity to predict protein structures based on primary amino acid sequences, informing the design of novel biomolecules with pharmacologically desirable properties. Combining rational molecular design with accessible high-throughput DNA synthesis will allow for rapid iteration and evolution of therapeutic enzymes or other biologically active proteins.

Another area where the ability to rapidly synthesize iterative DNA sequences is important, is in the development of DNA and RNA aptamers.6 Sometimes referred to as “chemical antibodies,” aptamers are 20–60-nucleotide oligomers that bind a range of targets from inorganic molecules to macromolecular complexes or even whole cells with high specificity and have significant diagnostic and therapeutic potential.
Aptamer development begins with a diverse library of synthetic oligonucleotides, which undergo rounds of evolution and selection for binding properties (SELEX). Access to rapid high-throughput on-demand DNA synthesis will therefore greatly speed up the process of aptamer screening and optimization.
Vaccines
Synthetic DNA is required in the development of many types of vaccines, including DNA, mRNA, and viral vector vaccines. As the COVID-19 pandemic has demonstrated, speed is of the essence when developing novel vaccines to cope with outbreaks and evolving variant strains. Rapid on-demand DNA synthesis combined with the new generation of vaccine platform technologies make it possible to go from the genetic sequence data of a novel pathogen to building a range of vaccine candidates for testing in a matter of days.
Although much attention has been focused on mRNA and viral vector vaccines due to their role in the pandemic response, there is renewed interest in DNA vaccines, driven by recent breakthroughs in delivery, immunogenicity, and sequence optimization.7 Furthermore, DNA vaccines are highly stable, removing the need for cold-chain shipping and storage, and are easier to make than mRNA or viral vaccines.
Not only are DNA vaccines currently being trialed in COVID-19 and other infectious diseases, there are also significant opportunities in oncology through the development of synthetic DNA vaccines designed to trigger an immune response tailored to the unique neoantigens expressed in each patient’s disease. Although the development of fully synthetic novel vaccines has been restricted in the past due to the challenges of synthesizing whole plasmids, the ability to generate highly accurate kilobase-scale synthetic DNA will open up exciting new possibilities in personalized cancer immunotherapy.
Conclusion
Synthetic DNA has numerous applications in drug development across a wide range of modalities, from library construction to novel biologics, vaccines, and more. However, its use has been hampered by slow, centralized services and a lack of accuracy in longer sequences. The coming generation of on-demand DNA synthesizers will bring unprecedented accuracy, scale, and speed, democratizing access to this technology and realizing the full promise of synthetic DNA in accelerating the development of tomorrow’s medicines.
References
1. Hughes RA, Ellington AD. Synthetic DNA Synthesis and Assembly: Putting the Synthetic in Synthetic Biology. Cold Spring Harb. Perspect. Biol. 2017; 9(1): a023812. DOI: 10.1101/cshperspect.a023812.
2. Saeed AFUH, Wang R, Ling S, Wang S. Antibody Engineering for Pursuing a Healthier Future. Front. Microbiol. 2017; 8: 495. DOI: 10.3389/fmicb.2017.00495.
3. Almagro JC, Pedraza-Escalona M, Arrieta HI, Pérez-Tapia SM. Phage Display Libraries for Antibody Therapeutic Discovery and Development. Antibodies 2019; 8(3): 44. DOI: 10.3390/antib8030044.
4. Zhu Z, Shaginian A, Grady LSC, et al. DNA-Encoded Macrocyclic Peptide Library. Methods Mol. Biol. 2019; 2001: 273-284. DOI: 10.1007/978-1-4939-9504-2_12.
5. Mullard, A. DNA tags help the hunt for drugs. Nature 2016; 530: 367–369. DOI: 10.1038/530367a.
6. Maier KE, Levy M. From selection hits to clinical leads: progress in aptamer discovery. Mol. Ther. 2016; 3: 16014. DOI: 10.1038/mtm.2016.14.
7. Gary EN, Weiner DB. DNA vaccines: prime time is now. Curr. Opin. Immunol. 2020; 65: 21–27. DOI: 10.1016/j.coi.2020.01.006.
Michael Daniels ([email protected]) is head of product management and Raquel Sanches-Kuiper is director of biology at Evonetix.