Immuneering prefers the hum of purposeful activity to mere noise. That helps explain why the company develops drugs to dampen the cacophony of cell signalling known as cancer.
To identify drug candidates, Immuneering uses its own Disease Cancelling Technology. Characteristically, the company has been quiet about the technology, which represents more than a decade’s worth of diligent effort. But now that the technology is ready, Immuneering is ready to talk about it in calm, assured tones.
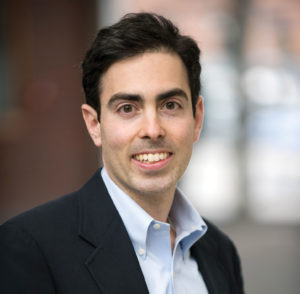
Co-Founder and CEO
Immuneering
“People talk about drug hunters, targets, and hits,” says Ben Zeskind, PhD, co-founder and CEO of Immuneering. “But for complex diseases, drugs actually have more complicated impacts, modulating hundreds of genes in ways that sometimes counteract the disease.”
That’s why focusing on the broad picture described by gene expression data can yield robust results faster and more effectively than traditional target development methods. It’s also more cost-effective.
Zeskind suggests that Disease Cancelling Technology is analogous to noise cancelling technology, which works by creating sound that is 180 degrees out of phase with whatever noise needs to be eliminated. Disease Cancelling Technology uses a similar approach, rating thousands of compounds and targets in terms of their ability to attenuate the disease signal at the level of human gene expression.
“Transcriptomics information shows that certain aspects of the disease change the gene expression profile,” he explains. “Tumors are an example. To metastasize, they must change their gene expression profile.” Disease Cancelling Technology is based on the principle that reversing those changes can counteract the disease.
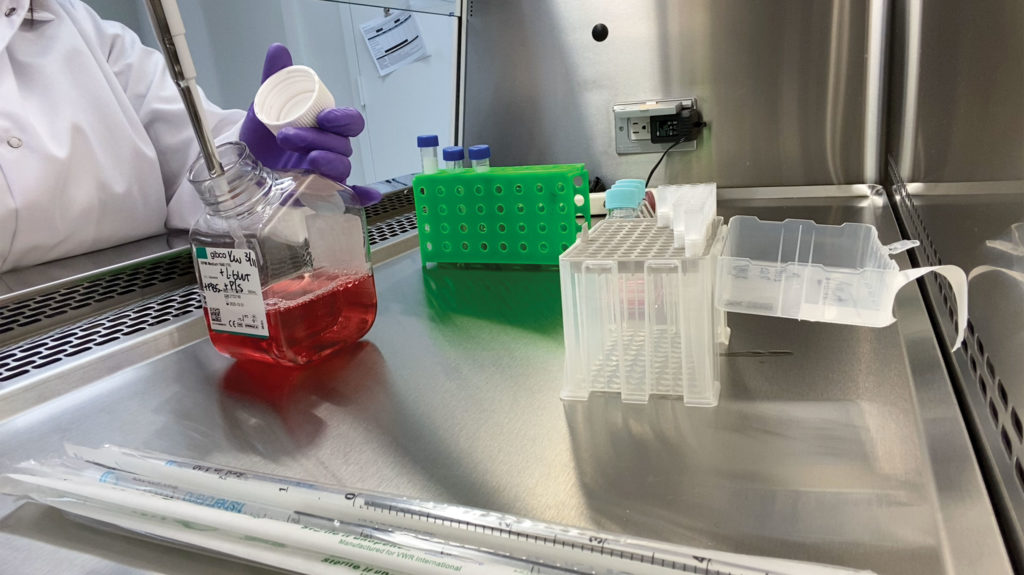
An unconventional approach
“Our platform produces a much steadier stream of output than traditional drug development,” Zeskind states. “As long as we have good human gene expression signatures, we will find novel insights. That is true for our chemistry platform, too.”
The choice of human clinical gene expression data coupled with chemistry is based on the challenges associated with traditional chemical or biological approaches, which usually try to identify targets in cell lines.
As Zeskind elaborates, “A cell line grown in a petri dish often does not reliably exemplify the disease across patients. Instead, it may mirror the disease in one, but not another. The cell lines used in pancreatic cancer research, for example, aren’t good genetic models for a lot of patients’ tumors.” Also, the three-dimensional nature of tumors, versus the two-dimensional nature of most lab models, reflects a different microenvironment.
That others haven’t developed this approach is a testament to Immuneering’s depth of experience in both bioinformatics and computational biology. “We’ve spent a decade doing this and have some two dozen scientific publications,” Zeskind points out.
Although biopharma companies are beginning to embrace artificial intelligence and machine learning, half those companies are purely chemistry based, Zeskind asserts. “They’re finding better ways to make better molecules for validated targets,” he continues. “Others are trying to find new biology using in vitro data. What makes us unique is that we can do both.
“We have both a biology and a chemistry platform, so we can find insights in new targets and create new molecules to drug those targets. That’s a rare combination. Our biology is based on human transcriptome data.”
“We’re focused on data reproducibility and quality control,” he declares. “That’s one of the least glamorous but most important things we do.” Immuneering has demonstrated its commitment to quality by having the company’s chief strategy officer serve as a board member of the Massive Analysis and Quality Control Society. This organization advances rigorous, reproducible scientific principles and promulgates quality control measures within the biopharma industry.
A history of quiet diligence
Immuneering was founded in 2008, around the time Lehman Brothers collapsed. Perhaps the ensuing market crash inspired Immuneering to value substance over bluff and bluster. Immuneering adopted a succinct philosophy: “Quit talking about it, and just do it!”
The company quietly built itself into a reliable service provider that helps its clients characterize mechanisms of action, identify targets and biomarkers, and analyze data from biobanks and clinical and laboratory experiments from single analytes to omics scale.
Then Immuneering took the insights it gained and launched itself as a drug developer in addition to being a service provider. “It was a very gradual process,” Zeskind recalls. “We bootstrapped ourselves for seven years, working with great pharmaceutical partners.”
In early 2016, the company began developing a discovery tool. “We started out looking to develop partnerships around the platform,” Zeskind relates, “but potential clients said, ‘If it’s so great, why don’t you use it yourself?’ We ran the numbers, gave it some thought, and decided to give it a try. It took us two years to get it exactly right.”
“We had built a race car, but we needed a driver,” Zeskind admits. So, the company hired Brett Hall, PhD, as CSO. Hall has extensive drug development experience, honed at Johnson & Johnson, MedImmune, and Asellus, and is integral to helping Immuneering develop its own pipeline of wholly owned assets.
With the addition of Hall, Immuneering began to operate as a drug developer while continuing its service operations, with a firewall between the two. “No data is shared,” Zeskind emphasizes. However, he allows that best practices are portable.
Promising leads
The company’s lead compound, IMM-1611441, targets the dual RAF-MEK pathway, which is overactivated in more than half of all human cancers. “Currently available drugs are relatively toxic and limited in their efficacy,” Zeskind notes. “So, we have found a way to drug that pathway—a way that, in preclinical data, is more durable and less toxic.” The first clinical trial is likely to launch within one year.
Immuneering also is developing IMM-2019611, a trifecta MEK currently at the lead optimization phase, along with several other drug candidates targeting KRAS, RAS, and other pathways. Early research suggests that a pan-KRAS approach may kill tumors driven by multiple RAS mutations. A neuroscience pipeline, focused on Alzheimer’s disease to identify new patient subsets, and an immuno-oncology pipeline also are being developed.
A business in transition
Last summer, Immuneering announced that its neuroscience division, Alleo Labs, will collaborate with Astex Pharmaceuticals, a wholly owned subsidiary of Otsuka Pharmaceutical, to identify therapeutic targets that counteract specific aspects of neurodegenerative disease. Besides pursuing partnership arrangements, Immuneering is embracing a new identity as a clinical-stage company by adding staff with clinical expertise.
Essentially, Immuneering is partnering with others and relying on its own internal programs to broaden the use of Disease Cancelling Technology. These actions, more than any words, speak to Immuneering’s confidence in its ability to reverse a disease signal across multiple relevant genes, and thereby treat cancer.
Similar aims may be pursued by Thermo Fisher customers. According to Hühmer, some of the company’s customers are taking advantage of the sophisticated signal amplification approaches offered by the Thermo Scientific Orbitrap Exploris 480 mass spectrometer and the Thermo Scientific Orbitrap Eclipse Tribrid mass spectrometer. These instruments, he says, can be used to “quantify more than 2,000 proteins from a single cell.”
Looking into the interactome
A single cell contains approximately 10 billion protein molecules that may be seen as interactive network elements, much like the 7.8 billion people on Earth may be seen as participants in a vast human social network. Although a cell’s proteomic network works on a tinier scale than the human social network, it might exhibit as much complexity.
In proteomic networks, proteins and protein complexes participate in dynamic, context-specific interactions locally and globally to support biological processes. Understanding these interactions could help scientists develop complex-selective therapeutics and clinical diagnostic methods.
“Identifying interactions in endogenous settings is difficult due to the inherent complexity of the protein population and the fleeting nature of their interactions,” admits Ray. “Two labeling strategies, covalent crosslinkers and proximity biotinylation, are allowing these interactions to be studied en masse with MS. Both labeling techniques allow scientists to tag those events within cells as they occur.”
An innovative bead-conjugation assay developed by PerkinElmer is being leveraged by the National Center for Advancing Translational Sciences, an arm of the National Institutes of Health, to support the search for effective COVID-19 therapeutics. With this assay, which is called AlphaLISA, light is emitted if a biomolecular interaction occurs in proximity of a bead-binding complex.
“Our proximity assays are [facilitating] antiviral drug repurposing research during the COVID-19 pandemic,” states Anis H. Khimani, PhD, senior strategy and market segment leader at PerkinElmer. They are helping to identify therapeutic compounds capable of disrupting the interaction between the SARS-CoV-2 spike protein and the human host receptor, ACE2.
Beyond mass spectrometry
Currently, proteomics relies mainly on MS, an approach that differs from initial proteomics approaches such as Edman degradation. Instead of truly sequencing, MS classifies enzymatically digested proteins based on the mass/charge ratios of protein fragments. Although MS-based proteomics matches genomics in terms of throughput and accuracy, it suffers in terms of sensitivity. Limited sensitivity and insufficiently long read lengths make it difficult for MS to detect rare proteins in mixtures containing abundant proteins.
In a recent review in Science Advances, Winston Timp, PhD, assistant professor at the department of biomedical engineering, Johns Hopkins, and Gregory Timp, PhD, professor, electrical engineering and biological studies, University of Notre Dame, note that in the near term, MS will likely be augmented by long-read transcriptomics and cellular indexing of transcriptomes and epitopes by sequencing (CITE-seq) or spatial transcriptomics, techniques that can simultaneously profile specific protein and nucleic acid signatures.
Alternative approaches include fluorescent fingerprinting methods, which can generate millions of single-protein reads using fluorosequencing by Edman degradation. In the longer term, the scientists predict that “once the kinks with throughput, bandwidth, and noise are worked out,” arrays of subnanopores will hold center stage in protein sequencing given their “extreme sensitivity and prospects for scaling.”
Therapeutics, biomarkers, and other applications
Proteomic signatures are providing diagnostic, therapeutic, and prognostic biomarkers. They are also deepening our understanding of basic biology.
“One area where we foresee a growing role for proteomics is structural biology,” comments Hühmer. “Ever since the detector revolution in cryo-electron microscopy, interest in this field has really been rekindled.”
Proteomics is leveraging the power of single-cell biology to point to novel ways of understanding how immunotherapies are creating more curative responses in patients. Examples from cell and gene therapy are offered by Mackay.
“We’ve been able to pick up proteomic signatures predictive of in vivo response, for instance, in CD19-oriented cell therapies, but also in novel preclinical cell therapies for solid tumor environments, which have traditionally been very difficult to treat,” he states. “We’re going to utilize the single-cell phosphoproteome to target the complete set of intracellular pathways to treat not only the first pathway that is engaged in a tumor cell, but also the pathways that cause resistance and metastases downstream.”
PerkinElmer is expanding its protein biomarker kit offerings across key disease and virus areas. The company’s assay for assessing the ACE2/S1 protein-protein interaction was described earlier. Other assays may advance development of cell and gene therapies, oncological therapies, and autoimmune therapies. “For downstream bioanalytical separation and analysis, we offer the LabChip® GX II for adeno-associated virus applications, as well as our new LC 300 UHPLC system with SimplicityChrom™ chromatography data system,” adds Khimani.
Quantitative proteomics is being used to support the discovery, characterization, and optimization of therapeutic monoclonal antibodies. The development of stable isotope–labeled antibodies has enabled researchers to use LC-MS/MS to characterize and optimize their biotherapeutics in new and innovative ways. For example, assays for pharmacokinetic studies in animal models can be developed in a much quicker fashion using LC-MS/MS without the extensive assay validation associated with immunoassays. MS-based studies can also provide insights into the mechanism of a drug’s degradation and clearance.
“We see increased use of stable isotope–labeled materials,” notes Ray. “We also see the need for certified reference standards in therapeutic drug monitoring studies to provide accurate measurements of clearance of these drugs in patients.
“Biomarker discovery and stratification will continue be a huge topic in the coming years, particularly in the identification of biomarkers to assess prognosis, and in the classification of responders versus nonresponders to therapeutics. We also expect to see more refined assays. They may include new and existing biomarkers and reflect an improved understanding of isoforms and post-translational modifications.”
Challenges
Advances in instrumentation and bioinformatics have widened the application of proteomics in basic research and in the development of clinical therapeutics. However, challenges remain in the automation of sample preparation, the standardization of analytical procedures, and the sharing of data (including data from reference datasets).
There is an unwillingness to accept the expenses associated with initial setup and maintenance, and a reluctance to adopt innovative technology in the development of therapeutics. All these challenges discourage the widespread use of proteomics in basic, translational, and clinical laboratories. If these challenges are not favorably answered, promising therapies will continue to be abandoned, and proteomics will be relegated to preclinical studies as a hypothesis-generating tool in select laboratories that can afford it. Democratizing proteomics technology will be pivotal in the coming decades.