“Why can’t we be more like salamanders,” said no one ever, except perhaps a regenerative medicine scientist. As the only larger vertebrate that can regenerate entire new limbs, these slithery, slimy biological marvels represent an inspiring example of what today’s scientists are seeking…regenerative superpowers. But we are not there yet.
Although we don’t understand the genetics enough to regenerate lost limbs and other tissues, we are making great strides engineering the technology to replace them. Researchers must first build the methodologies and technologies to produce the appropriate tissues. Whether creating biological inks for 3D printing or constructing a compatible architecture for scaffolds, next-generation biomanufacturing tools are making headway to drive this fledgling industry from bench to bedside.
The Bioprocess International Conference, to be held next month in Boston, is featuring a pre-conference symposium highlighting next-generation tools for manufacturing regenerative medicine products. Speakers will describe the use of microcarriers in scalable bioreactors to produce billions of cells, new 3D fabrication processes that can exactly recapitulate the size and shape of needed tissues, and how to create an assembly line for automating tissue production.
Billions and billions of cells
Human mesenchymal stem cells (MSCs) dominate regenerative medicine as the most utilized cell type. Harvested from bone marrow or adipose tissue, they provide the critical raw material for a variety of applications, from cancer and stroke treatments to tissue regeneration. Although MSCs can differentiate into a number of cell types, including chondrocytes (cartilage), myocytes (muscle cells), osteoblasts (bone cells), and adipocytes (fat cells), expanding them can take weeks or months.
According to Taby Ahsan, PhD, senior director of development at RoosterBio, there are more than 1000 ongoing clinical trials employing MSCs. She explains, “Trials often require up to a billion cells per patient. Thus, there is a critical need to produce cells derived from a consistent process that yields a standardized MSC product. To do this requires that we change from the old paradigm of utilizing cells with variable passages and population doublings to a new process using more consistently defined products.”
With an eye toward the future, RoosterBio has begun pursuing “next-gen” MSC bioprocessing strategies. “Expanding cells on microcarriers in a scalable suspension bioreactor system, we routinely now generate 25–30 billion cells in a single development run. This same system can currently be directly scaled by at least 10–20-fold,” she discloses. “Meanwhile, we are continuing to optimize our processes to further improve our cell expansion rates and yields for consistent production for the GMP arena and clinical uses.”
Ahsan says RoosterBio is also helping at-the-bench researchers overcome current production challenges. “Our approach is to provide affordable, thoroughly characterized cells, at a much larger initial volume, that gets the researchers to their experiments in a faster and more consistent manner. Helping to save on time and costs allows researchers to further advance science and technology with the same resources.”
For example, the company guarantees that cultivating its “Research Use Only” bone marrow–derived MSCs with its bioprocess media systems will result in a 10-fold expansion to over 100 million cells within one week.
Bioprocessing of MSCs, in particular, is a field on the move. Ahsan predicts, “With their potential to treat so many diseases, we expect continuing improvements in MSC bioprocessing will drive production to unprecedented levels of rigorously prepared clinical products that are primed and ready to go.”
Next-gen tissue bioreactor
Our bodies consist of 206 bones held together by 306 joints, all of which will erode over a lifetime. Additionally, bone damage or loss may result from traumatic injuries, congenital defects, or illness. Why not use our own adult stem cells to provide the starting material to grow new bone and joints? That was the question posed when EpiBone began operation in 2014.
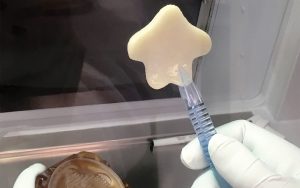
“Our team has developed a way to grow new bone from a patient’s own stem cells, and we hope to use this technology to restore patients to full form and function,” reports Sarindr “Ik” Bhumiratana, PhD, EpiBone’s CSO and co-founder. To accomplish this task, the company engineered and optimized its bioreactor technology to create optimal bone growing conditions, so that they mimic the natural conditions found in the human body, providing appropriate nutrients and oxygen as well as necessary biophysical cues.
The three-step process to create new bone begins with a CT scan that generates an anatomically precise 3D picture of the area to be replaced or repaired, which is then used to design an anatomically precise scaffold. “Our 3D fabrication process matches the exact curvature, shape, and cutting angles to perfectly fit the patient’s needs,” says Bhumiratana.
In the second step, a patient’s adipose tissue stem cells are isolated and expanded. These cells are grown in an appropriate medium and seeded onto the scaffold in the proprietary perfusion bioreactor. In the third step, it takes only a couple of weeks for the cells to proliferate and differentiate into osteoblasts, which create bone tissue ready for implantation, according to Bhumiratana.
The company’s approach can overcome many of the current limitations in the field, he adds. For example, it can avoid allograft rejection, prevent impermanent integration (as seen with plastics and metal), and eliminate the multiple surgeries that are necessary when harvesting bone from the patient’s body.
Another challenge in the field is scaleup. “Scalability is a challenge in the regenerative medicine space, but we are actively incorporating automated technologies that could exponentially increase our manufacturing capabilities, at a fraction of the original cost,” projects Bhumiratana.
The company is currently gearing up to commence its first Phase I/II trial on its lead bone product, EpiBone Craniomaxillofacial (EB-CMF), in patients with mandibular ramus continuity defects.
Biocompatible building blocks
While the methods and materials utilized to engineer biomaterial scaffolds vary greatly, one common component is collagen. As the main structural component and building block of the extracellular matrix, collagen excels as a natural biocompatible material for regenerative medicine. By fine-tuning its structure via manufacturing processes, collagen-containing scaffolds can be created for myriad applications, including ones for soft tissue, orthopedics, advanced wound care, and cardiovascular repair.
“We are an FDA-registered company that produces medical devices and components and that manufactures specialized biomaterials as high-quality sheets, films, powders, gels, or bio-inks needed for building scaffolds,” explains Peter Gingras, chief executive officer, Viscus Biologics.
According to Gingras, special care must be taken when biomanufacturing starting materials, such as those for tissue and organs. He points out, “Producing biocompatible components and inks for creating scaffolds can be challenging. It is important that collagen and other native polymer biomaterials be specially purified with minimal manipulation, yet still be able to provide the inherent features needed for each application.”
Some applications require different types of collagen as well. The company provides custom services for clients who need specific concentrations and forms. Gingras expects that next-generation biomanufacturing tools for regenerative medicine products will see some important changes: “I believe the future will see an increasing emphasis on the creation of high-performance materials that are scalable yet cost-effective, as well as GMP compliant.”
Manufacturing innovations
Accomplishing the goal of widespread use of tissue-engineered medical products (TEMPs) depends on the fulfillment of one overarching mandate. “It’s time to focus on manufacturing,” declares Jennifer Briggs Braswell, PhD, chief administrative officer of the Advanced Regenerative Manufacturing Institute’s (ARMI’s) BioFabUSA program. “Development of the industry for TEMPs calls for scalable processes and manufacturing lines that are modular, automated, and closed.”
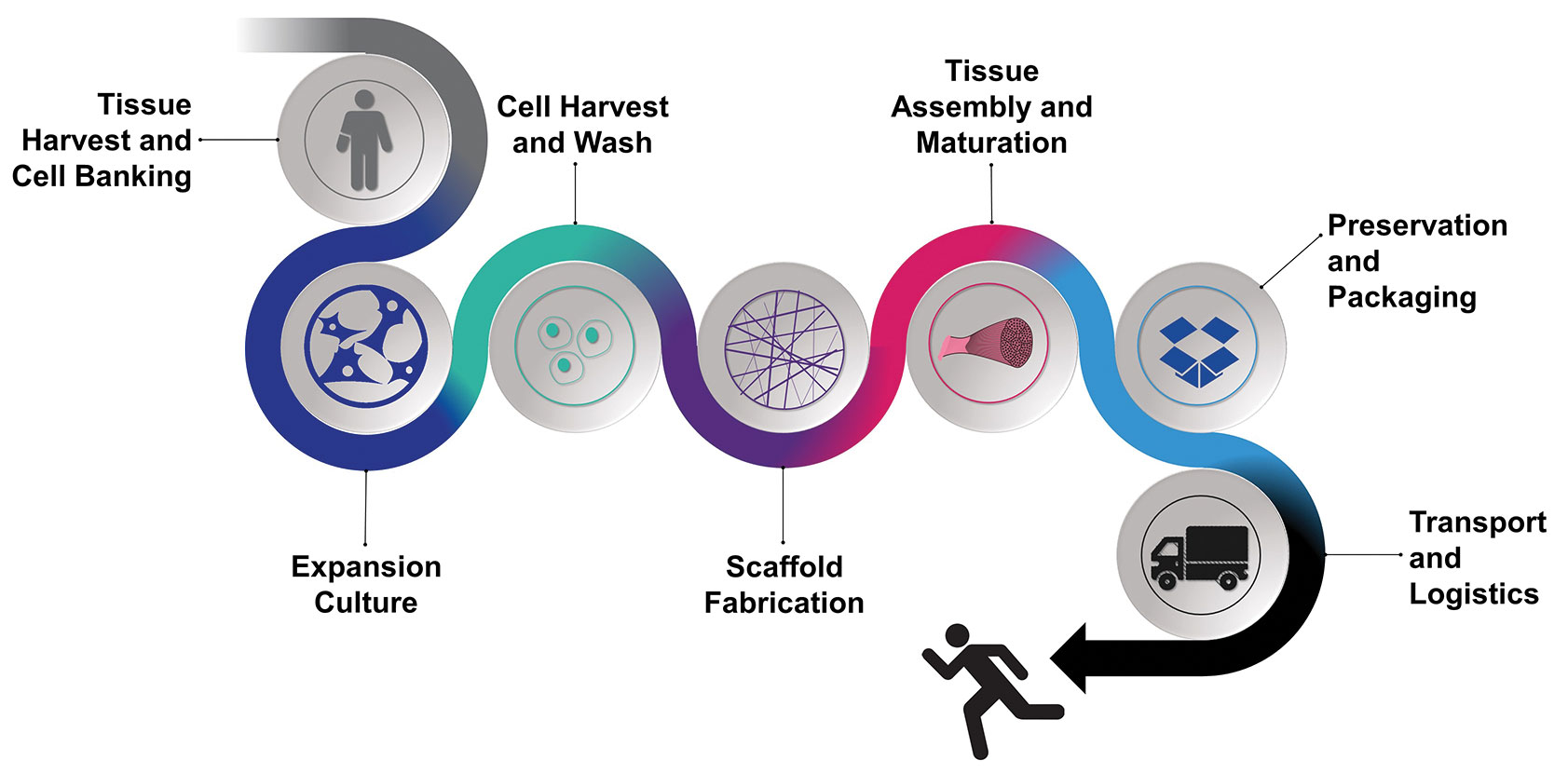
Braswell says that BioFabUSA member institutions and companies are collaborating to advance manufacturing: “For example, BioFabUSA has demonstrated the Tissue Foundry, a prototype manufacturing line that uses the concepts of modular manufacturing to group unit operations into five modules: cell culture and expansion, harvest and wash, scaffold fabrication, tissue assembly and maturation, and preservation and packaging.” Ultimately, the manufacturing line will be GMP compliant.
According to Braswell, a lesson of the last 10 years is that future manufacturing improvements will drive commercialization and expansion of the industry: “In order to further develop the field, we need to attract investment capital. In the past, TEMP manufacturing seemed too complicated and too costly. I believe that manufacturing improvements will be a driver of the cost reductions needed to draw investors and more widely advance TEMPS.”