Even before COVID-19 emerged, virologists and other scientists worried that the flow of new and improved human viral vaccines had slowed to a trickle. Vaccines remained unavailable for viral diseases caused by familiar pathogens (such as human immunodeficiency virus, hepatitis C virus, and most herpesviruses), as well as for viral diseases caused by emerging pathogens (such as avian influenza, Ebola, and Zika viruses).
In September 2019, just a few months before the current pandemic, the state of vaccine development and manufacturing was the subject of a review article that appeared in the Journal of Virology. The review’s authors, led by the Burnet Institute’s Gregory A. Tannock, PhD, DSc, noted that in previous decades, vaccines against polio, measles, mumps, rubella, and hepatitis B viruses became available. It was a golden age. Then, after 1990 or so, relatively few new vaccines progressed to licensing. The decline, according to Tannock and colleagues, might have reflected the scarcity of “low-hanging fruit,” the economic constraints faced by profit-driven operations, and the lack of substantial government intervention.
Today, less than a year and a half after this somber assessment, vaccine development and manufacturing has been reinvigorated, largely due to the urgency imposed by COVID-19. Late in 2020, two COVID-19 vaccines—one from Pfizer and BioNTech, and one from Moderna—were approved for emergency use authorization. Many others are in development and clinical trials.
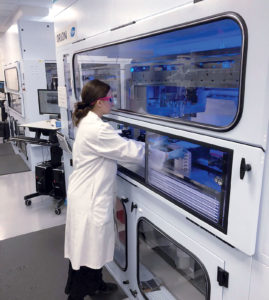
According to Charles Christy, head of commercial solutions, Ibex Dedicate, Lonza, the response to the COVID-19 pandemic has been driving progress with several vaccine formats including inactivated vaccines, nonreplicating vaccines, adjuvanted recombinant protein vaccines, DNA and mRNA vaccines, live attenuated vaccines, protein subunit vaccines, T cell–based vaccines, and more.
It is clear that the COVID-19 emergency is speeding vaccine development. It also appears that vaccine development may gain enough momentum to overcome new challenges once COVID-19 is contained.
Recent advances
Of the new formats, mRNA vaccines are especially interesting, according to Christy. They offer significant potential advantages in terms of speed, platform production, and dose sparing. With these advantages, mRNA vaccines could facilitate the rapid treatment of global populations.
Speed was certainly evident during production of the Moderna COVID-19 vaccine (mRNA-1273). This vaccine went from sequence selection to preclinical evaluation in 63 days. Also, just 10 months after the sequencing of SARS-CoV-2 (the virus that causes COVID-19), mRNA-1273 had entered commercial production.
Moderna, which has entered a strategic collaboration with Lonza to manufacture up to 1 billion doses annually, currently has 23 mRNA development candidates with 12 in clinical studies across a wide range of indications.
“New advancements in mRNA vaccines and other novel vaccines may mean that we are entering a new golden age for vaccines,” says Christy. “However, important work remains to ensure global access, adequate capacity, and ease of administration. In addition, the industry has a key education task to ensure widespread uptake.”
Extra benefits
“The first demonstration that RNA-based vaccines can protect you against infectious disease is clearly a major advance in vaccine development and production,” says Philip Dormitzer, MD, PhD, vice president and CSO, Viral Vaccines R&D, Pfizer. The RNA-based vaccine against COVID-19 developed by Pfizer and BioNTech reflects several technological choices. The partners eschewed biological living substrates, made use of an enzymatic process to produce the RNA, and took advantage of structure-based protein design to stabilize the antigen in its prefusion conformation.
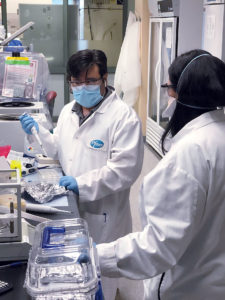
Structure-based antigen design also showed that the key antigen used in previous respiratory syncytial virus (RSV) vaccines was in the wrong conformation. A structurally designed Pfizer maternal RSV vaccine with higher immunogenicity is currently in a Phase III trial.
In the pandemic’s wake, advances in vaccine development have been paralleled by advances in vaccine manufacturing. Typically, manufacturing and scale-up investment is withheld until a proof-of-concept study has demonstrated a vaccine’s efficacy. Due to the vast societal need, Pfizer invested in manufacturing infrastructure while the company was still engaged in research and development. All the while, Pfizer maintained the same quality standards it applies during normal vaccine development.
Lessons learned during the development of COVID-19 vaccines could be applied to the reengineering of legacy vaccines, says Dormitzer, especially those most in need of performance improvements. Such legacy vaccines include flu vaccines.
From year to year, the flu vaccines vary in efficacy depending on how well they match the flu viruses in circulation. “In a good match year, the vaccine will provide 60% efficacy, which is far from the 95% we are seeing with the COVID-19 vaccine,” notes Dormitzer. “In mismatch years, the efficacy can be as low as 10–20%.”
“We would love to have an 80% effective vaccine,” he continues. “We think some of the RNA technologies can be brought to bear. With these technologies, you can change the vaccine to better match the changes going on in the virus without having to change processes. You need only minimal changes to assays.”
RNA technology elicits not only antibodies but also a strong T-cell response. By increasing valency and adding T-cell immunity to antibody-mediated immunity, it may be possible to increase the breadth of the flu vaccine so that every vaccine strain covers a greater range of the circulating viruses.
If RNA technology is to be exploited to the full in countering influenza, it may be necessary to accommodate the different capabilities possessed by national influenza laboratories. Worldwide, there are over 130 national influenza laboratories. They constitute a large network. However, some of the laboratories may be better positioned than others to collect, analyze, and share the kinds of information needed by developers of RNA vaccines.
Change drivers
Effective vaccines are still needed against many older viruses such as Marburg virus, Lassa virus, RSV, cytomegalovirus, Epstein-Barr virus, and chikungunya virus. Effective vaccines are also needed for many newer viruses. Since 1980, scientists have identified more than 80 emerging viruses including H5N1 virus, H1N1 virus, human rhinovirus species C, MERS-CoV virus, and Nipah virus. On average, two novel viruses are identified each year. Despite recent successes—for example, the Ebola vaccines VSV-EBOV and AdVac/MVA-BN—new vaccines need to be generated more quickly.
One way to expedite vaccine development is to exploit rational vaccine design. According to Christy, rational vaccine design is now driven by bioinformatics, massive advances in sequencing speed and capabilities, and a better understanding of immunology.
Vaccine manufacturing can also realize benefits, such as improved yields and more robust processes, from enhancements in bioreactors and fermenters, closed systems, and purification processes. And enhanced analytical methods can help ensure safety, identity, purity, and potency.
Cell-based vaccine manufacturing technology is replacing older technologies, such as egg-based production technology. Cell-based technology can help accelerate and scale up processes while allowing for simultaneous production of multiple viral vaccines. In addition, processes based on cell-based technology can incorporate insect cell cultures, DNA technology, and mRNA technology to generate improved vaccines.
The implementation of new technologies and improved processes requires significant investment, as does the expansion of vaccine production infrastructure and manufacturing capacity. Global pre-investment by governments and organizations will need to be reinforced by ongoing investment if there are to be effective responses to future epidemics/pandemics.
Besides exposing weaknesses in vaccine manufacturing, the COVID-19 crisis has also revealed the need to expand manufacturing capacity for monoclonal antibodies. Existing manufacturing capacity for monoclonal antibody treatments was already highly utilized before such treatments were deemed suitable for use against COVID-19.
Manufacturing workflows
New manufacturing platforms that have shown promise include viral vector vaccines that use genetically engineered viruses, such as adenoviruses, to transport genetic information that will code for a disease antigen within the body. An example of a viral vector vaccine is Ervebo, the Ebola vaccine that was approved by the FDA in 2019.
In addition, the emerging mRNA platforms are providing agility to vaccine manufacturing. Because these platforms are modular and agnostic with respect to disease target, they allow vaccine variants to be generated and evaluated without requiring the manufacturer to modify or revalidate production processes. This is a massive change for an industry that usually dedicates one building or production area to one vaccine. Because viral vector and mRNA platforms are still maturing, their optimization and refinement remains important.
“We are equipped to manufacture products for all platforms, including viral vaccines, viral vector vaccines, mRNA and pDNA vaccines, and recombinant protein vaccines,” says Amélie Boulais Raveneau, head of market entry strategy, Viral-Based Therapeutics, Sartorius. “We provide flexible solutions for vaccine manufacturing because we never know exactly what the market needs will be.”
Flexible solutions often incorporate single-use technologies, which have grown significantly in popularity. Single-use technologies allow implementation and validation to be accomplished more quickly than is possible with traditional stainless-steel technologies, which require strict cleaning protocols. Single-use technologies promote flexibility by allowing facilities to pivot quickly to new modalities. Switching to single-use products also enables sustainability; retrofitting facilities for other modalities prevents future waste and reduces carbon footprints.
“The simpler mRNA manufacturing process makes it easier to manufacture locally for streamlined distribution to a wider range of areas,” adds Boulais Raveneau. “We have seen this pandemic underscore the need for localized supply chains to allow manufacturers to mitigate their risk if disruption occurs in a certain region.”
In addition, most of the frontrunners in the race for a COVID-19 vaccine demonstrate the critical advantage of ensuring collaboration and communication among all parties including developers, manufacturers, and regulatory bodies.
However, Boulais Raveneau believes that there is still a long way to go to make workflows more efficient in general. For example, process intensification refers to protocols that enable manufacturers to cut down on time, steps, input material, and costs. Although this idea has been around for a while, she anticipates a wider implementation. Along the same lines, Bioprocessing 4.0—the automation of manufacturing workflows—will become increasingly important as manufacturers strive to improve efficiency.
Oral vaccines
Just-in-time delivery is advantageous for production and distribution facilities of all sizes, including the tiny ones known as living cells. For example, living cells can be used to generate mRNA and encapsulate it in virus-like particles. Thus packaged, the mRNA may be shipped to other cells, including the body’s cells, which accept delivery of the mRNA and use it to guide production of key viral proteins.
Unlike DNA cargo, which may be integrated into the host genome, mRNA cargo limits genotoxic risks. However, mRNA is inherently unstable. When used as a vaccine format, mRNA poses challenges with respect to synthesis, stabilization, packaging, and delivery.
“We have adopted mRNA as a core technology,” says David O’Hagan, PhD, co-founder, president, and CEO, Esperovax. “[We] deliver it orally to reach the intestines, which house 70% of the body’s immune system. Our vaccines work in situ. We synthesize the mRNA moments before it reaches the small intestine immune cells, which then present the patient-built proteins/antigens to the immune system.
“Our proprietary oral vaccine approach should alleviate many of the limitations and logistical challenges faced by mRNA vaccine producers. And taking a pill or gummy is less intimidating than being poked with a needle. We expect this approach will lead to safer, more effective vaccines, and that it will reduce the fear of the vaccination process.”
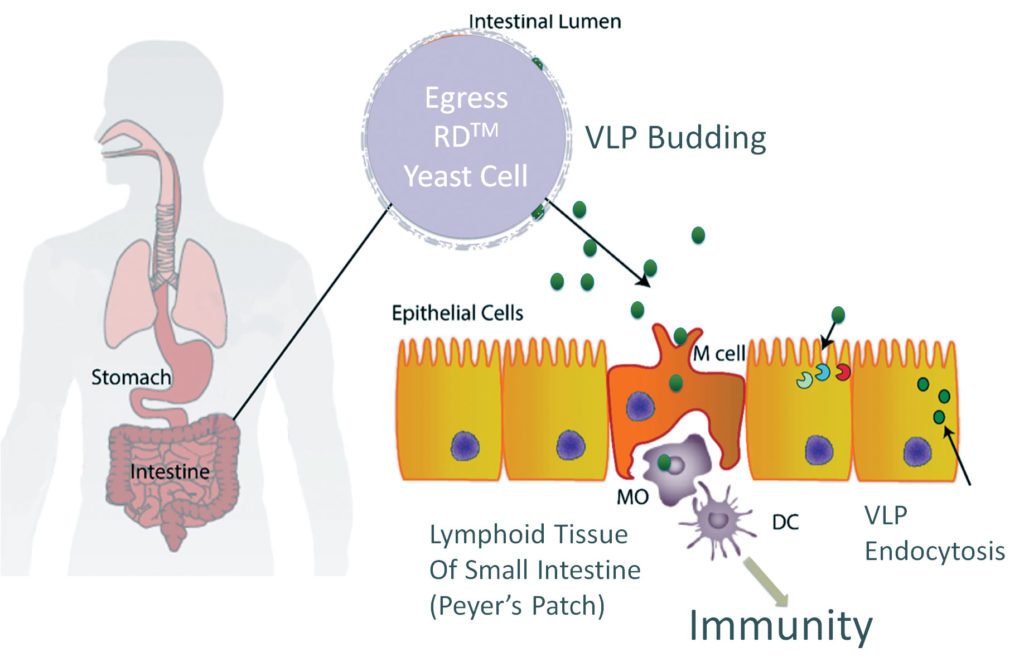
Esperovax uses Saccharomyces cerevisiae, a yeast species that has been instrumental in winemaking, baking, and brewing since ancient times, as an inexpensive way to deliver RNA to cells that are naturally designed to receive that signal. “By just using the same amount of yeast that brewers make in a day, we could vaccinate the entire world’s population 68 times,” observes O’Hagan. “The ability to rapidly produce vaccines that are shelf stable and based on mRNA would be a significant advancement.”
The mRNA does not need to be protected from degradation. The yeast produces the RNA-containing nanoparticles seconds before use. A built-in switch prevents the cells from dividing, eliminating contamination of the broader ecosystem.
The company currently has a contract from the Biomedical Advanced Research and Development Authority to test an oral COVID-19 vaccine in mice and hamsters. The delivery technology could allow a rapid global response to any pathogen. Increases in population, in travel, and in food animal transport have exacerbated the evolution of new strains of pathogens. Once the COVID-19 pandemic passes, emphasizes O’Hagan, the world population must remain vigilant and invest heavily in protective measures against future diseases.