It is 21 years since the death of teenager Jesse Gelsinger nearly obliterated the field of gene therapy. In 1999, Jesse received a dose of the ornithine transcarbamylase gene, engineered into a recombinant adenovirus, at the University of Pennsylvania. The idea was for the gene to zero in on liver cells. However, soon after the treatment, he developed jaundice, inflammation, and multiple organ failure. Within four days, Jesse was dead.
Since then, genetic technology has made gigantic leaps forward. The human genome has been sequenced in its entirety, and the cost of sequencing has plummeted 100,000-fold. CRISPR-Cas9 has emerged as a precise and efficient tool to edit genes. Artificial intelligence and deep learning are being put to use in designing molecules. And gene therapy has morphed from a blacklisted experimental concept to a life-altering practical solution for a growing list of previously incurable diseases.
To date, the U.S. Food and Drug Administration (FDA) has approved five gene therapy products for a half-dozen diseases (Table). Earlier this year, the FDA announced that it “anticipates many more approvals in the coming years, as there are more than 900 investigational new drug applications for ongoing clinical studies in this area.”
The ideal target for gene therapy is a Mendelian disease caused by defined single-gene mutations, such as hemophilia or Duchenne muscular dystrophy. That has been its initial focus, bolstered by approvals of drugs such as Luxturna (for a type of Leber’s congenital amaurosis, an inherited blindness disorder) and Zolgensma (for spinal muscular atrophy).
Now, gene therapy is taking on more complex, multigene diseases such as central nervous system diseases, neuropathic pain, sleep apnea, and cancer. Beyond correcting genes, gene therapy is revolutionizing cell-specific delivery of therapeutic proteins and synthetic drugs, as well as inspiring basic research into unanswered questions on immunogenicity. But lest anyone think gene therapy has solved all of its earlier issues, the deaths last summer of two young boys in an experimental trial for a rare muscular disorder remind us that the field still has a lot of work to do to ensure gene therapy can be safely delivered for all patients.
Delivery
The first order of business is to deliver therapeutic gene products into target cells. Vehicles used to transport genes into the cell include genetically modified viruses for long-term genomic integration or lipid nanoparticles for more transient tasks such as expressing genome editing nucleases.
The reason for gene therapy’s healthier outlook in 2020 is “improvements in the technology of gene transfer,” says James M. Wilson, MD, PhD, director of the Gene Therapy Program at the University of Pennsylvania. “Our work with adeno-associated viruses and the work of a number of other investigators, including that of Luigi Naldini, MD, PhD, in creating lentiviral vectors, were fundamental advances that paved the way for everything else.” He credits the clinical successes of his Penn colleague Jean Bennett, MD, PhD, on retinal blindness; the work of Nationwide Children’s Hospital’s Jerry R. Mendell, MD, on spinal muscular atrophy; and “the pivotal work on hemophilia out of St. Jude’s.”
The former vector favorite, adenovirus, has been largely supplanted by adeno-associated viruses (AAVs), generally used for in vivo gene therapy, and lentiviruses, used to modify cells outside the body, such as chimeric antigen receptor (CAR) T cells in the treatment of cancer. These ex vivo engineered cells are then amplified in tissue culture and returned to the patient.
AAV-based gene therapy has made great strides in recent years. Pioneering work from the lab of Terence R. Flotte, MD, dean, provost, and executive deputy chancellor of the University of Massachusetts Medical School, has propelled clinical trials for two classic Mendelian disorders, alpha-1 antitrypsin (AAT) deficiency and Tay-Sachs disease. With excellent safety and durability established in a five-year follow-up of its Phase IIa trial, the AAT gene therapy is now in its final investigational new drug–enabling toxicology study stage.
“AAT trials have shown some unusual tolerogenic properties,” says Flotte. “The study shows patients develop regulatory T cells against AAV coat proteins that prevent them from reacting negatively even without pharmaceutical immune suppression.”
Nearly 75% hemophilia patients take regular intravenous infusions of a recombinant factor VIII (F8) protein coupled with other drugs that prevent bleeding or the breakdown of clots. “Current treatment for hemophilia A is very burdensome to patients,” says Bettina M. Cockroft, MD, chief medical officer at Sangamo Therapeutics.
A new therapy being developed by Sangamo and Pfizer injects a recombinant AAV6 encoding F8, which could provide a permanent solution. “The gene therapy for F8 shows lasting activity,” Cockroft reports. “Five patients tested in Phase I/II of the clinical trial did not need F8 infusions and had no bleeding events.”
Vectors injected into blood generally don’t get across barriers. Wilson’s lab, in collaboration with Amicus Therapeutics, is focusing on developing penetrating next-generation AAVs that deliver more efficiently to cells in organs such as the muscle, heart, and brain. “With the new AAVs,” notes Wilson, “you can get more delivery with less vector. Less vector would mean less toxicity.”
Outlook
Patient and public support for gene therapy is crucial for its advance. “I have a pretty good idea of how stakeholders view gene therapy,” Wilson asserts. “Because gene therapy is primarily focused on treating and potentially curing diseases of significant unmet need that are disabling or lethal, I see incredible enthusiasm for us to move forward.” Public and private ventures have invested heavily in the promise of curing genetic disease by altering or replacing faulty genes.
The change in the academic, industrial, and public outlook on gene therapy is readily appreciable in the reaction to the recent tragic deaths of two young boys in a gene therapy trial, sponsored by Audentes Therapeutics, for X-linked myotubular myopathy (XLMTM), despite impressive efficacy in preclinical studies, as discussed in a recent episode of GEN Live (“Gene Therapy: What’s Up With AAV?”). Instead of blacklisting the entire field of gene therapy as was seen decades earlier, the trial has been put on temporary hold while researchers reevaluate data so that such fatalities can be avoided in the future.
“Audentes has elaborated further on the data surrounding the deaths in a recent letter to Human Gene Therapy,” says Flotte, the journal’s editor-in-chief. The presence of comorbidities, particularly liver disease, and prior exposures to the virus in these patients might have played a role, in addition to technical challenges, such as the lack of standardized assays for measuring levels of preexisting viral antibodies. Ongoing preclinical investigations are focused on establishing safer doses and understanding immune mechanisms in response to AAV that might exacerbate existing liver dysfunctions.
“In the long term,” says Nicole Paulk, PhD, assistant adjunct professor, biochemistry and biophysics, UCSF, “we need to shift our focus from ‘How can we safely use high doses?’ to ‘How can we design the vector so we don’t have to?’” Limiting the viral dosage necessary for maximal efficacy in gene therapies is being evaluated through experimental approaches, such as engineering more penetrating capsids, introducing regulatory elements for tissue-specific viral expression, developing better manufacturing and purification processes to increase the purity of virus injected, and devising strategies to avoid immune responses.
More than correcting genes
Drug delivery:
Future applications of gene therapy technology for more common, polygenic diseases will not necessarily treat defective genetic components directly. Instead, these applications may improve the delivery of proteins that are otherwise used to treat diseases. An example is RGX-314,a developed by RegenXbio, for the treatment of neovascular age-related macular degeneration, where unwanted blood vessels form in the retina that result in blindness. This gene therapy uses a NAV AAV8 vector that encodes a vascular endothelial growth factor (VEGF)-neutralizing monoclonal antibody fragment. A one-time subretinal injection of RGX-314 is enough for the sustained synthesis of anti-VEGF therapeutic protein and circumvents the need for repeated intravitreal injection VEGF-neutralizing proteins.
Modifier gene platform:
Ophthalmologist Mohamed Genead, MD, chair of Ocugen’s retina scientific advisory board, is excited about a revolutionary new approach—the modifier gene platform—that takes a “gene-independent approach” by overexpressing upstream regulatory genes for nuclear hormone receptors (NHRs) in retinal cells instead of attempting to correct mutations in individual genes.
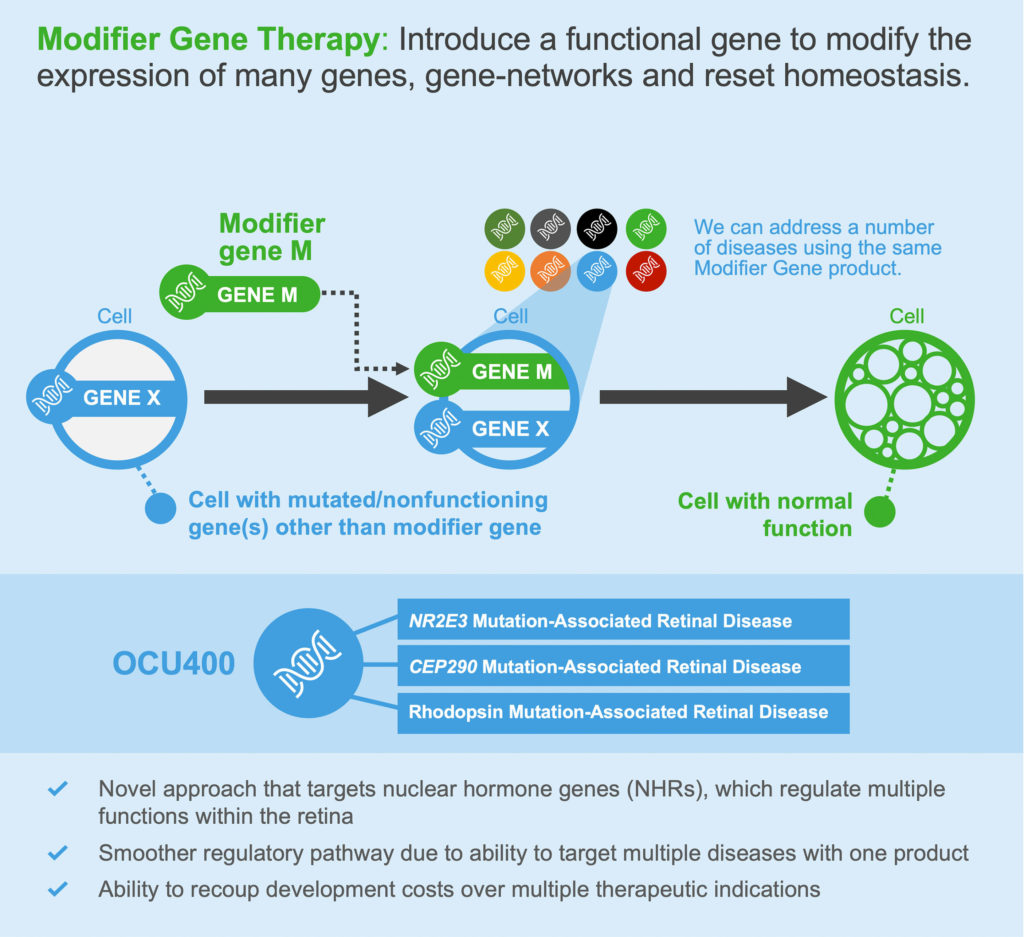
“Overexpressing NHRs does not correct the gene mutations; instead, it corrects the disease phenotype,” says Genead. “Conventional gene therapy has several limitations. The amount of DNA you can package into the virus is restrictive. Gene therapy can work if you have identified the mutation, but most genetic diseases do not have identified mutations.”
NHR overexpression regulates endoplasmic reticulum stress to promote normal function in cells with defective genes. “Its efficacy remains to be shown in the clinical trials,” comments Genead, “but if we see in patients what we see in animal models, there will be a significant shift of the treatment paradigm in patients with retinal degeneration.”
Chemogenetic therapies:
Continuous positive airway pressure machines or surgery are the currently available treatments for obstructive sleep apnea, a condition where the tongue loses muscle tone and blocks the upper airway in sleeping patients. Consequences range from fatigue and metabolic disruptions to stroke and death.
An alternative approach is being explored by researchers at Johns Hopkins Medical School. They are evaluating whether DREADDs—designer receptors exclusively activated by designer drugs—can be used to activate the genioglossus muscle, and thereby improve breathing during sleep.

“We are inserting designer receptors for synthetic drugs in specific brain cells to maintain patency of the airways,” says Thomaz Curado, MD, PhD, research associate at Johns Hopkins. “If translated successfully in human clinical trials, this can mean a one-time injection combined with a nightly pill will eliminate risk of death due to obstructive sleep apnea.”
Conferring resistance to infectious diseases:
Single-gene disorders and infectious diseases are being treated using the same tools and concepts. “We are in the midst of a very aggressive program in using AAV as a way to prevent infections of COVID-19,” says Wilson. Essentially, AAV vectors are, as usual, being used to engineer cells in patients to express proteins. In this program, however, the proteins confer resistance to COVID-19.
AAVCOVID, a gene-based vaccine that will deliver genetic sequences of the SARS-CoV-2 in an AAV capsid, is being developed jointly by the Gene Therapy Program at the University of Pennsylvania and the Massachusetts Eye and Ear and Massachusetts General Hospital, and it is scheduled to enter clinical trials later this year.
Safety, success, and staying on
Lacunae in the basic understanding of how the immune system responds to foreign vectors are currently at the forefront of investigations on toxicity and inflammation induced by gene therapy.
“What factors predispose complement activation and the hemolytic-uremic syndrome at high-doses of rAAV? What factors predispose to inflammation in the dorsal root ganglia and spinal cord after direct rAAV-mediated gene delivery into the central nervous system? What is the nature of liver toxicity in patients treated with high doses of rAAV gene therapy? [These] are the frontier questions,” says Flotte. “How significant are immune responses to Cas9 and other nucleases? How important is the toxicity from off-target gene editing effects? [These] are questions facing gene editing in the context of gene therapy.”
One of the limitations of the ubiquitous AAV vector has been the mounting of an antibody response against the vector itself, according to Federico Mingozzi, PhD, chief scientific officer at Spark Therapeutics. Hence the interest in imlifidase (IdeS), an endopeptidase that degrades circulating antibodies without disrupting B lymphocytes that produce antibodies. The enzyme, already in the clinics for transplantation patients, has two main benefits for gene therapy. It can be used to treat seropositive patients, and it permits the re-administration of the gene therapy product if the need arises.
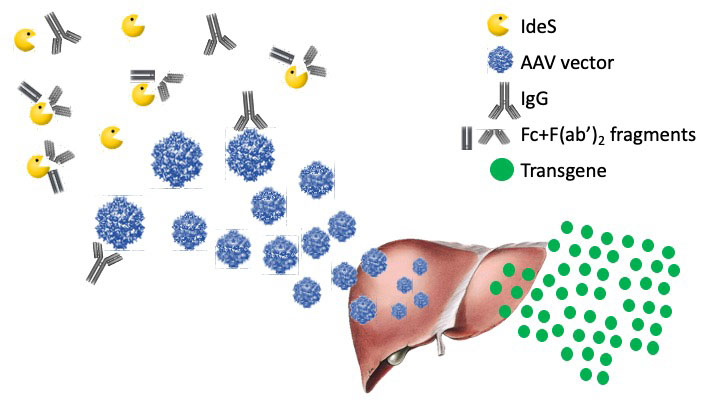
“Once you give IdeS, you see a sharp and immediate drop in circulating antibodies. They stay low for 3–4 days and then go up again,” says Mingozzi. “Of course, it has to be tested in humans in conjunction with gene therapy, but it has a very good safety profile.”
“The reality is that we are at the beginning of a revolution,” declares Wilson. “The forward move for gene therapy is to make it safer and more effective.” Making it durable depends on the context but depends on three key factors: The genome must be stable, the cell has to persist, and therapeutic gene expression cannot be turned off. “Of these three,” says Wilson, “I think the major challenge is going to be to assure that its expression is ongoing.”
“Durability is a major consideration for rAAV gene therapy and is intertwined with immune and inflammatory toxicity,” adds Flotte. “It is less of a concern in gene editing, which involves permanent changes in the genome.”
Accessibility
According to Laura Hercher, director of student research, Human Genetics Program, Sarah Lawrence College, “the main roadblock is going to be price and accessibility. Unfortunately, in countries where there isn’t that kind of money to spend on a single patient, it is only going to be available to the very wealthy.”
A new nonprofit called the Institute for Life Changing Medicines aims to secure, develop, manufacture, and distribute life-changing medicines such as gene therapy at cost. “Ultimately, we want to provide access in countries where the diseases might not be rare, but the governments who are responsible for reimbursements simply won’t pay,” says Wilson. “I’m going to do everything I can to the last day I’m on my job to assure [global access]. What we are trying to do to assure global access is to be very efficient in clinical development and manufacturing to contain cost.”
Following a No Disease Left Behind strategy, the nonprofit is currently committed to providing gene therapy solutions for Lesch-Nyhan syndrome, aromatic L-amino acid decarboxylase deficiency, and Crigler-Najjar syndrome type I—diseases where gene therapy has a proven transformative effect.
aThe original text left the mistaken impression that RegenXBio produces Lucentis. The text has been modified to make clear that RegenXBio produces RGX-314. Lucentis (also known as ranibizumab) is a similar but not identical anti-VEGF produced by Genentech/Roche.