CRISPR systems that rely on inactivated Cas enzymes—that is, dead Cas (dCas) enzymes—never looked more alive. They harness the targeting power associated with CRISPR—but not the double-strand cuts. As such, they give researchers new ways to interrogate and manipulate gene function.
Possibilities include CRISPR interference (CRISPRi) and CRISPR activation (CRISPRa) systems, which bind to specific DNA sequences and thereby modulate the expression of targeted genes. They use a dCas fused with a transcriptional repressor (in the case of CRISPRi) or a transcriptional effector (in the case of CRISPRa).
Additional possibilities include CRISPRoff and CRISPRon, which are also means of modulating gene expression. However, they combine dCas9 with DNA-altering enzymes that add and remove methyl groups, respectively. The epigenetic effects of CRISPRoff are lasting, even multigenerational, but they can be reversed by CRISPRon.
CRISPR systems that incorporate dCas fusion proteins may be used in combination with one another or alongside non-CRISPR-based techniques, such as RNA interference, to validate results orthogonally. Interrogating gene function with multiple techniques, each using a different cellular process, tackles the constant challenge of data reproducibility head-on.
In research that explores disease mechanisms and potential treatments, there are additional means of boosting data confidence. For example, this kind of research can incorporate sophisticated models such as differentiated induced pluripotent stem cells (iPSCs), primary immune cells, or organoids. These models are desirable because they may be used to capture the nuances of disease. However, they can be challenging to work with in combination with CRISPR systems.
The expanding array of dCas9 tools and the challenges of using CRISPR systems in concert with sophisticated cell models were discussed in a recent digital event hosted by The CRISPR Journal and GEN. The event’s participants—five leading researchers in the areas of CRISPR and stem cell research—presented insights that deserve the widest possible circulation. Accordingly, we summarize them here, in this GEN supplement article.
Expanding the CRISPR toolbox
In his opening keynote presentation, Stanley Qi, PhD, an assistant professor of bioengineering and chemical and systems biology at Stanford University, outlined how the basic CRISPR-Cas9 toolbox has expanded, particularly with his invention of dCas9 to create an RNA-guided genome targeting system.

The still-growing family of dCas tools now includes dCas9, dCas12, and dCas13. Those tools, in turn, have been put to use in a wide range of research applications such as gene regulation with CRISPRi/a, epigenome editing, chromatin imaging (LiveFISH), and spatial genomics (CRISPR-GO).
Given the urgency of the COVID-19 pandemic, Qi and his collaborators translated dCas9 technology to develop CRISPR antivirals using dCas13. “In 2019, we were inspired by a question of how antivirals can be designed in weeks instead of months,” Qi recalled. His group’s work on a CRISPR-dCas13-based strategy called PAC-MAN (Prophylactic Antiviral CRISPR in huMAN cells) was timely enough to use as a prototype system for development of an antiviral against SARS-CoV-2, as well as influenza A.
Qi’s group chose two highly conserved regions of the coronavirus genome to target: the regions for RdRp and nucleocapsid. RdRp and nucleocapsid are much more highly conserved than spike protein, the more common target of vaccines and antivirals. In a lung cell model, the CRISPR RNA (crRNA) targeted against the viral RdRp and nucleocapsid regions showed 98% inhibition of live SARS-CoV-2.
This approach not only promises to be a very effective treatment for SARS-CoV-2, but it may also combat whole families of viruses, according to Qi. He noted that just six crRNAs can target more than 90% of all coronaviruses. This raises the possibility of designing a single CRISPR-dCas13 antiviral agent with pancoronavirus activity.
Long-lasting analgesia
Pain is a major area of unmet need worldwide and particularly in the United States, where 30% of Americans live with chronic or severe pain, according to Ana Moreno, PhD, CEO of Navega Therapeutics. Moreno presented her company’s work on long-lasting analgesia via targeted in situ repression of a new pain target, NaV1.7.
“Around 30% of Americans live with some sort of chronic or severe pain,” Moreno said. “These patients rely mostly on opioid narcotics, but these have really failed us. In the past decade, there’s been a 2% increase in prescriptions without any change in reported pain. One in four patients that are prescribed opioids become addicted to them.”
The NaV1.7 ion channel is encoded by the SCN9A gene. A loss-of-function mutation of SCN9A leads to a congenital insensitivity to pain. There is also a gain-of-function mutation that causes primary erythromelalgia, a type of autosomal dominant neuropathy characterized by episodic pain.
Building on this biology, Moreno speculated that NaV1.7 could be an entirely new target for pain relief and an alternative to opioids, the drugs that have led to an epidemic of addiction in the United States. Subsequent research into NaV1.7 informed the development of an epigenetic gene therapy targeting pain that is nonaddictive, highly specific, and long lasting.
Navega’s therapy uses dCas9 plus KRAB, a transcriptional repression domain from zinc finger proteins, to repress NaV1.7. The company is also developing a similar therapeutic using zinc fingers. The therapies are designed to be delivered in an adeno-associated vector, AAV9, via intrathecal injection.
Because dCas9 has no cutting activity, there are no permanent changes to the genome, which would lead to the undesired effect of permanent loss of ability to feel pain.
In a mouse model of inflammatory pain, animals that received the therapy had higher pain thresholds than control animals. Moreno shared that it’s exciting to be able to build on previous work with dCas9 and zinc finger nucleases to develop solutions for patients with chronic and severe pain. She emphasized that “nonaddictive gene therapy for these patients would be life changing.”
Translating genome editing technologies
Shondra Pruett-Miller, PhD, is the director of the Center for Advanced Genome Engineering at St. Jude Children’s Research Hospital. For her presentation, Pruett-Miller outlined a novel fitness assay (for CRISPR knockout screen validation) and ongoing work on a CRISPR gene therapy approach for hemoglobin SC disease (a hemoglobinopathy similar to sickle-cell disease).
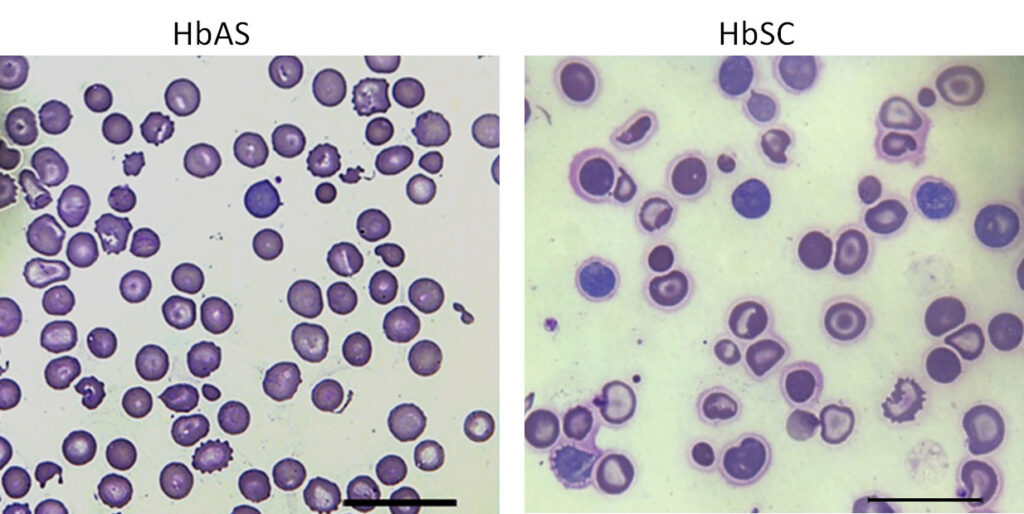
After covering best practices for pooled CRISPR screens, Pruett-Miller described her fitness assay. She said it starts like a typical validation assay, with Cas9 plus a guide RNA, and is delivered as ribonucleoprotein via nucleofection to the cells. The cells are then transfected transiently with ribonucleoprotein, and the genomic DNA is harvested at several time points. The region of interest is amplified by PCR and submitted for targeted next-generation sequencing.
The assay becomes a validation assay for CRISPR screening at the next step, when the resulting in-frame and out-of-frame indels are separated and followed over time. That analysis shows a clear pattern of out-of-frame indels decreasing over time when an essential gene is knocked out.
For translation of gene editing into the clinic, Pruett-Miller aimed for the “low-hanging fruit” of gene therapy: stem cells in the blood. These cells can be removed from the patient, edited, and reinfused. Her team focused on hemoglobin SC disease, a hemoglobinopathy in which patients inherit one copy of the hemoglobin S variant, and one hemoglobin C variant.
When Pruett-Miller used CRISPR-Cas9 with base editing to correct the mutation leading to hemoglobin C, nearly 20% of the cells returned to wild-type hemoglobin. Her group has also developed a hemoglobin SC mouse model to test whether the edited cells can cure the disease. “Our next experiment,” Pruett-Miller indicated, “will be to do a bone marrow transplant into an irradiated mouse to see if we can rescue the pathophysiology of hemoglobin SC.”
Pruett-Miller concluded that CRISPR screening is a powerful tool for functional genomics, but she also advised the webinar’s attendees that “your results are only as good as your model, your assay, and your perturbation.”
Brain disease “risk in a dish”
Most of the risk for schizophrenia resides in about 250 loci, each of which confers a 1% increased risk of schizophrenia. That’s according to Kristen Brennand, PhD, a professor of psychiatry and genetics at the Yale School of Medicine. In her presentation, Brennand outlined how gene editing contributed to her work using stem cells to study brain diseases, particularly schizophrenia. Her research combines functional genomics, advanced CRISPR techniques, and stem cell models to investigate illnesses that result from complex effects of genes and environmental factors on different cell types and circuits in the brain leading to disease.
Brennand’s laboratory is working to understand the synergistic effects of schizophrenia risk variants and to determine which genes and pathways would make suitable therapeutic targets. CRISPR gene editing and CRISPRi/a were key tools her team used to study five expression quantitative trait loci in schizophrenia that had been previously identified through genome-wide association studies (GWASs). And of those, one locus near the furin gene was a prime choice to begin gene editing.
“It’s the only time in the entire GWAS,” Brennand pointed out, “where the same SNP that’s most significant for schizophrenia risk is also the same SNP that’s regulating the nearby target gene.”
This work is being done in a human-induced pluripotent stem cell (hiPSC)-based neuroprogenitor cell (NPC) model derived from patients with schizophrenia. Neurons grown from those cells are similar to fetal neurons. But schizophrenia is a disease that manifests much later in life, so the cells cannot be seen as a model of the disease.
“These are not disease-in-a-dish models,” Brennand insisted. “Rather they’re disease-risk-in-a-dish models. We’re modeling predisposition to disease, not the disease itself.”
Brennand and colleagues used CRISPR gene editing to validate a potential causative SNP in the furin gene, and with CRISPRi/a, they were able to validate three other multi-SNP candidate genes.
In further studies, Brennand and colleagues found that manipulation of schizophrenia common variant expression affects function, and that there were unexpected synergistic effects between those variants. “Manipulating only 4 of the 250 risk genes in combination, we’re already seeing emergent biology,” Brennand reported. “And so, I think it behooves us as a field to move toward larger and larger combinations so that we can begin to really test how these risk genes are summing when inherited together.”
Changing epigenetic memory
Luke A. Gilbert, PhD, assistant professor of urology at the University of California San Francisco (UCSF) School of Medicine, closed the event with a keynote presentation on writing and erasing epigenetic memories. Specifically, he described CRISPRoff, a tool that establishes gene-silencing DNA methylation marks. Introduced in a recent paper (Nuñez et al. Cell 2021; 29; 184: 2503–2519), CRISPRoff uses a dCas9 fusion protein to programmably target any protein domain to any sequence of DNA in human cells. The paper also discussed a complementary tool called CRISPRon. It removes methylation marks deposited by CRISPRoff, making the epigenetic manipulation process fully reversible.
“What my lab is focused on,” Gilbert said, “is turning gene expression on and off for single genes and sets of genes and the whole genome essentially, and then using readouts such as cell function or fluorescence or survival to understand how single genes and sets of genes contribute to complex phenotypes and how genes act in coordination.”
He pointed out that although some of the same objectives can be accomplished through genome editing, when it comes to therapeutic applications, double-stranded DNA breaks can be toxic, and DNA repair can be unpredictable. Gilbert posited, “If all you want to do is turn a gene off, perhaps there are more straightforward ways to do that using epigenetic editing.”
CRISPRoff is based on the cell’s natural DNA methylation system, which it uses to repress endogenous retroviruses. Building on previous work in the literature showing that KRAB combined with the DNA methyltransferases DMNT3A and DMNT3L could induce gene silencing when recruited to a target locus, Gilbert’s team set out to combine those three elements in a single protein. The result? The CRISPRoff fusion protein.
The CRISPRoff platform uses transient transfections of guide RNA and CRISPRoff constructs to seed a stable, repressive mark at the target gene, and then monitors gene silencing over time. Gilbert asserted that silencing continues stably for about 50 days in a variety of cell types, and that it is highly specific and heritable.
Gilbert concluded that CRISPRoff is a robust platform for heritable gene silencing for a majority of human genes. This platform, he elaborated, is compatible with high-throughput CRISPR screening and likely to advance the exploration of chromatin biology. He also indicated that the platform has potential applications across therapeutics and personalized medicine as well as in cell and tissue engineering.