Anything that is worth doing—including cell therapy manufacturing—is worth doing imperfectly at first. Refinements can wait. In the meantime, cell therapies can benefit patients who lack other options. Also, any improvements that are made on the fly can contribute to an iterative development cycle. If cell therapy manufacturers can maintain a flight path while development proceeds, there’s no telling how far they might go.
Cell therapy products that generate impressive early-phase clinical trial data may be cleared for takeoff by the U.S. Food and Drug Administration select FDA). For example, the FDA approved Novartis’ Kymriah select tisagenlecleucel) for children and young adults with refractory or relapsing acute lymphoblastic leukemia. The agency based its decision on evidence of high remission rates in three Phase I/II studies. Likewise, the FDA approved Gilead’s Yescarta select axicabtagene ciloleucel) for adults with large B-cell non-Hodgkin lymphoma based on data from a Phase I/II trial. In this trial, called Zuma-1, a remission rate of 51% was achieved.
For some therapies, early approvals—that is, approvals based on early clinical trial data—make sense, particularly if alternative treatments do not exist. Early approvals also present production opportunities. These opportunities, however, come with potential downsides. For example, early approvals may give manufacturers little time to hone their production processes. For hard-to-make products such as cell therapies, commencing large-scale manufacturing sooner rather than later can keep production costs high.
High cost of goods
“In recent years, cell therapy approvals have been driven by a tailwind of terrific clinical data,” says Anthony Davies, PhD, founder and CEO of Dark Horse Consulting. “This has thrust commercial licensure on complex products which have spent a relatively short amount of time in pharmaceutical development. Approvals out of, effectively, Phase II trials are becoming likely for products with even less than Phase II–grade chemistry, manufacturing, and control select CMC) readiness.”
According to Davies, underdeveloped processes increase the cost of goods as a result of the manual steps and the bespoke technologies used. Underdeveloped cell therapy processes also lack scalability, observes Fabio Fachin, PhD, head of cell therapy engineering and automation, Takeda.
Designing for scalability is paramount, Fachin insists, because high manufacturing costs slow the adoption of cell therapy products, even when they can boast impressive clinical outcomes. Also, the timelines between safety trials and pivotal studies for cell therapies are drastically shorter than those for biologics.
“A lean, scalable manufacturing scheme that can be mapped from early-stage to pivotal-stage clinical trials with minimal modification” offers significant advantages, Fachin explains. It can favor repeatability and controllability select and therefore easier analysis of clinical data and correlations with product specifications) as well as financial viability at the commercial stage. “This is where automation can provide a clear benefit,” he declares. “It can enable predictable workflows and sourcing requirements and even streamline QA/QC.”
Clinical-scale technology
The paucity of bioprocessing technologies also increases cell therapy manufacturing costs. Moreover, the most of the available bioprocessing technologies are intended for trial-scale rather commercial-scale production.
“The commercial cell therapy industry is indeed early in development,” says Scott R. Burger, MD, principal, Advanced Cell & Gene Therapy. “[It] tends to use tools developed for academic clinical trials, rather than scalable, highly efficient automated production.”
Technologies needed by the industry include those capable of supporting scalable processes, informatics applications, and the provision of specialized raw materials. Fortunately, technologies to serve the industry are beginning to emerge in response to burgeoning demand, which is due to the industry’s rapid growth. For example, as Burger notes, technology companies are increasingly focused on automating closed-system cell processing and informatics.
Automation
Fachin also sees automation as key. He suggests that developers will need to switch from manual processes if they intend to overcome their processing challenges. “The aim of cell therapy manufacturing is to deliver a consistent product starting from an intrinsically variable starting material, often from a diseased patient,” he says. “That is a big task.”
“So far,” he points out, “the cell therapy industry has relied on largely manual or at best semiautomated processes.” Going forward, however, a real inflection will be needed in the industry’s ability to deliver the right product for each patient. This inflection, Fachin insists, will occur “only when manual processing variability is removed from the equation and when full automation will be in place to optimize each manufacturing run” for the requirements posed by individual patients.
According to Fachin, automation should be considered and applied to all parts of cell therapy production—processing, analytics, and data management. He addresses each point in turn:
- Processing: Remove the manual operator component and transition to fully controlled automated operations, ensuring consistent if not identical performance lot to lot.
- Analytics: Transition to automated high-throughput, multiparameter assays that can be integrated in-line within the manufacturing scheme, so as to provide real-time information to control the automated processing tools.
- Data management: Transition to an infrastructure where data can be stored and then used not only to deliver correlations with patient outcomes, but also to provide predictions that could enable the real-time optimization of each product lot while it is being manufactured.
Automation is, in fact, becoming more common in process development and analytical method development. According to Fachin, both areas have been the focus considerable investment and technology development. In contrast, automation in data is less advanced.
“The use of big data and in-line correlative sciences in manufacturing is still at its inception, particularly in the autologous space,” he observes. “We are still far from having systems that allow us to refine the manufacturing process from lot to lot based on the specifics of the material at hand.”
Fachin notes that Takeda has invested and continues to invest in a vision where processing, analytics, and data management—the “three pillars”—come together: “Besides immediately deploying automated process and analytical platforms in our manufacturing processes, we are building robotics and data infrastructure tools and expertise that we believe will eventually enable us to deliver fully optimized, fully personalized drugs, independent of indication.”
Outsourcing dynamics
Automation and other forms of technological innovation are starting to change how cell therapies are made. However, most cell therapies are still produced by contractors.
In cell therapy manufacturing, as in industry generally, outsourcing is used to lower costs. But for cell therapy firms, there are often other factors at play. For example, according to Davies, the granting of early approvals is a major outsourcing driver.
“Partly due to the rapid developmental trajectory of many recent cell therapy approvals, many companies have simply not had time, money, or expertise to develop in-sourced manufacturing solutions,” he explains. “A small number of legacy CMOs, who have been in the field since before its recent tailwinds, have performed a large amount of manufacturing.”
Burger also acknowledges the pivotal role that contractors play. “The majority of cell therapy manufacturing is outsourced to contract manufacturers at present,” he says. “This is especially true early in development. There are several full-service cell therapy contract manufacturers like Lonza, Miltenyi Biotec, Cognate BioServices, Oxford Biomedica, Paragon Gene Therapy [part of Catalent Biologics], KBI Biopharma, MaSTherCell, Brammer Bio [part of Thermo Fisher Scientific], Cobra Biologics, and Hitachi Chemical Advanced Therapeutics Solutions—to name just a few.”
But the situation is changing thanks to technology and process innovation. “Better capitalized cell therapy companies are now commissioning significant in-house manufacturing capability,” Davies points out. “And some are hedging by utilizing this in combination with outsource options.”
Cell therapy capacity
Iovance Biotherapeutics is an example of a firm that maintains in-house cell therapy manufacturing capacity. In this case, the firm produces a range of tumor-infiltrating lymphocyte select TIL) therapies.
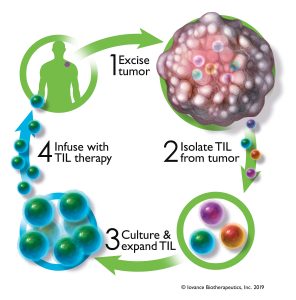
“TIL therapy is a cell therapy treatment that uses a patient’s own cancer-fighting immune cells to attack solid tumors,” says Arvind Natarajan, PhD, Iovance’s vice president of CMC. “TILs are extracted from tumor tissue. Once TILs are expanded and infused back into the patient, they should be able to seek and enter a tumor, where they recognize, attack, and destroy the tumor.”
To manufacture TILs, Iovance uses a process known as Gen 2. The process begins, Natarajan points out, when biopsies are removed from patient tumors. Next, the biopsies are shipped to a manufacturing facility, where the TILs are extracted and expanded. Finally, the TILs are returned to the patient.
“Our rapid Gen 2 manufacturing process allows us to complete the entire TIL manufacturing process in 22 days,” Natarajan asserts. “The cells are cryopreserved at the end of the manufacturing process, allowing flexibility in the timing of the infusion based on patient requirements.”
“The original Iovance process is licensed from the National Cancer Institute,” he notes. “We have since made significant improvements in the process. The current Gen 2 process is proprietary, so the specific process and applications are protected by the company’s intellectual property rights.
Iovance is investing $75 million over three years to build a custom commercial manufacturing facility in Philadelphia. According to Natarajan, the facility has a modular design to allow for scalability of production depending on necessary volume.
Cell therapy production regulations
Besides technological change, the cell therapy industry is experiencing regulatory change. According to Davies, regulators are being forced to seek out expertise and update requirements. He notes that reforms adopted by Japan’s Pharmaceuticals and Medical Devices Agency have rippled through the Asia-Pacific region, and that actions taken by the Gottlieb-era FDA have contributed to significant progress.
“On the one hand, the sheer volume of submissions has left regulators scrambling to find headcount to provide timely review,” Davies states. “But on the other hand, the technical complexity and novelty of the products makes such human capital hard to find, especially as qualified personnel are also being actively recruited by well-capitalized companies crafting the regulatory submissions.”
This view is shared by Burger, who says, “Regulations for cell therapy and gene therapy products are actually very well developed at this point, particularly in the United States, Europe, and much of Asia.”
Common standards
In cell therapy manufacturing, regulatory development is still in progress. To make this point, Fachin contrasts the cell therapy field with more mature fields. In mature fields, he says, industry standards are well established, whereas in cell therapy, regulatory agencies such as the FDA, the European Medicines Agency, and the Pharmaceuticals and Medical Devices Agency “are indeed still working with academic and industrial institutions to outline cell therapy standards.”
And according to Fachin, all parties agree that collaborating to set common standards will help industry to improve how cell therapies are made: “Recently, I had the privilege of leading a workshop on tool integration at Phacilitate Automation SIG, where representatives of cell therapy manufacturers, device manufacturers, regulators and, raw materials manufacturers came together. A unified session outcome was the fact that democratic academic/industry/regulatory engagement to define cell therapy ‘standards’ select at the hardware, software, device interfaces, manufacturing, analysis, and release levels) is a must for long-term scalability and sustainability.”
Pall and Recursion Collaborate on Human Umbilical Vein Endothelial Cell Scaleup
Efficiently growing cells—whether for cell therapy, drug screening, or process development—is crucial to the pharmaceutical business. Crucial, too, is assuring that those cells consistently maintain the attributes critical to their utility, sometimes even beyond standard single-assay measures.
Recursion Pharmaceuticals and Pall Biotech collaborated on a pilot project to transfer expansion of human umbilical vein endothelial cells select HUVECs) from standard spinner flasks to the Pall Allegro™ STR stirred tank bioreactor platform. “At Recursion, we’re basically doing drug discovery and development at scale. We’re trying to build a really large data set of these different drug screens, and we need to produce a lot of primary cells to accomplish those,” says Dylan Knutson, an HTS biomedical engineer at the company. He presented the results of the collaboration at last month’s Informa Cell and Gene Therapy Bioprocessing and Commercialization conference in Boston.
Adherent HUVECs were grown on collagen-based microcarrier beads. Those expanded in the bioreactor doubled significantly faster, reached confluence a day earlier, and showed significantly better viability upon freeze-thaw than those in spinner flasks. Both methods allowed the cells to maintain critical quality attributes select CQAs union for example, as demonstrated by the formation of tubules in the presence of vascular endothelial growth factor in a traditional angiogenesis assay.
“But then we took it a lot further,” Knutson explaines. Standardly grown cells were screened with 90 compounds from various drug classes at three different concentrations and stained with the multiparametric fluorescent Cell Painting assay, allowing them to be analyzed across more than a hundred features with Cell Profiler software. These data were used to train a machine learning algorithm to classify different phenotypes and correlate them with treatment. This was used to successfully predict, for example, the class of compound in an assay with bioreactor-generated cells.
“We get a really robust measure of function and performance,” Knutson says, adding that this approach can ensure the consistency of these features in every batch of cells for their sensitive assays.