Since the term “biological marker” was introduced roughly seven decades ago, biological markers, or biomarkers, have become more numerous. And partly through the sheer power of numbers, biomarkers have transformed research, clinical medicine, and public health. But biomarkers have more going for them than numbers. They also have context.
Biomarkers may, to some extent, contextualize each other if they are deployed in multiplex panels. Biomarkers may also be seen in spatial contexts if analyses preserve tissue morphology and incorporate high-resolution imaging. This kind of contextualization can be enriched further with other kinds of information, suggests Gavin Gordon, PhD, vice president of clinical market development, Akoya Biosciences.
“Many people equate biomarkers with DNA, RNA, or protein,” says Gordon. “But a biomarker can also encompass more complex characteristics such as data collected from an X-ray, enzymatic activity, or histologic analysis.”
Additional kinds of contextualization are being evaluated by biomarker developers. For example, these developers are demonstrating that biomarker information can be integrated with bioinformatic, proteomic, or gene signature information to uncover distinctive, disease-related patterns. Now that biomarkers are becoming more numerous and more contextualized, they promise to become increasingly intertwined with drug discovery and patient stratification, as well as with the development of novel predictive, diagnostic, or prognostic clinical tools.
Spatially resolved biomarkers
Initially, the biomarker field focused on the development of biomarkers that were one-dimensional. The typical biomarker was a single molecule whose presence, absence, or levels conveyed information about a biological process. More recently, the field has shifted toward identifying biomarker signatures that incorporate the spatial data of cells expressing relevant molecular markers.
“The complexity of these higher-order biomarkers reflects the complexity of the diseases they help unravel, particularly in immuno-oncology,” says Niro Ramachandran, PhD, chief business officer, Akoya.
Many biomarkers that interrogate biological samples are limited by the fact that they usually provide aggregate measures about information that is diffusely spread out across tissues. “That is a proxy for not having the right tool to look at spatially resolved biomarkers,” says Ramachandran. Studies have assessed how well biomarkers may predict patient responses to checkpoint inhibitor therapies. These studies suggest that simply assessing the presence or the absence of a molecule is insufficient to make informed clinical decisions.
“Many people who are biomarker positive don’t respond to a checkpoint inhibitor, and a meaningful subset of individuals who do respond are biomarker negative,” explains Gordon.
The paradigm developed at Akoya, rooted in many studies that have documented the importance of preserving spatial architecture during tissue analysis, is to develop spatially resolved biomarkers that can capture how cells of different phenotypes are distributed in a tumor sample. “How these cells organize and talk to each other can tell us how a patient will respond to therapy,” says Ramachandran. “That is the crux of studying tumor biology across multiple dimensions—individual cells, what markers they co-express, where they are located, and the impact on the microenvironment surrounding them.”
Akoya has developed Phenoptics™, a high-throughput platform for quantitative pathology and translational biomarker research. Phenoptics integrates multiplex immunohistochemistry and imaging to quantitatively to collect systems biology data while preserving cellular and spatial details. “This technology enables us not only to discover tissue biomarkers, but also to use them for clinical decisions,” says Ramachandran.
Akoya says that its technology can be used to develop phenotypic biomarkers; that is, the technology can help researchers interrogate cellular phenotypes and understand how they interact to generate tissue phenotypes. A key challenge of collecting such high-dimensional images is that every marker contributes exponentially to the size and complexity of the dataset. To help address the need for rapid image acquisition, management, and secure data sharing, Akoya recently announced the launch of Proxima™, a spatial biology analysis platform that can be accessed from any location and promises to reshape digital pathology.
“When you combine the latest advances in digital pathology and artificial intelligence with new technologies that examine tissues at unprecedented scale, you create a perfect storm,” says Ramachandran. “You strengthen biomarker discovery efforts that can bring us closer to curing cancer.”
Between cell enrichment and microscopy
“In all the clinical areas—but especially in oncology—the biomarker side of things is the least developed part of the field,” says Alan Schwebel, PhD, president and CEO, BioView. For two decades, BioView has been at the forefront of developing, manufacturing, and marketing cell imaging and analysis solutions for research and clinical biomarker applications. Recently, BioView scientists integrated microscopy techniques with an analysis algorithm to help characterize biomarkers that can unveil morphological and functional characteristics of circulating tumor cells.
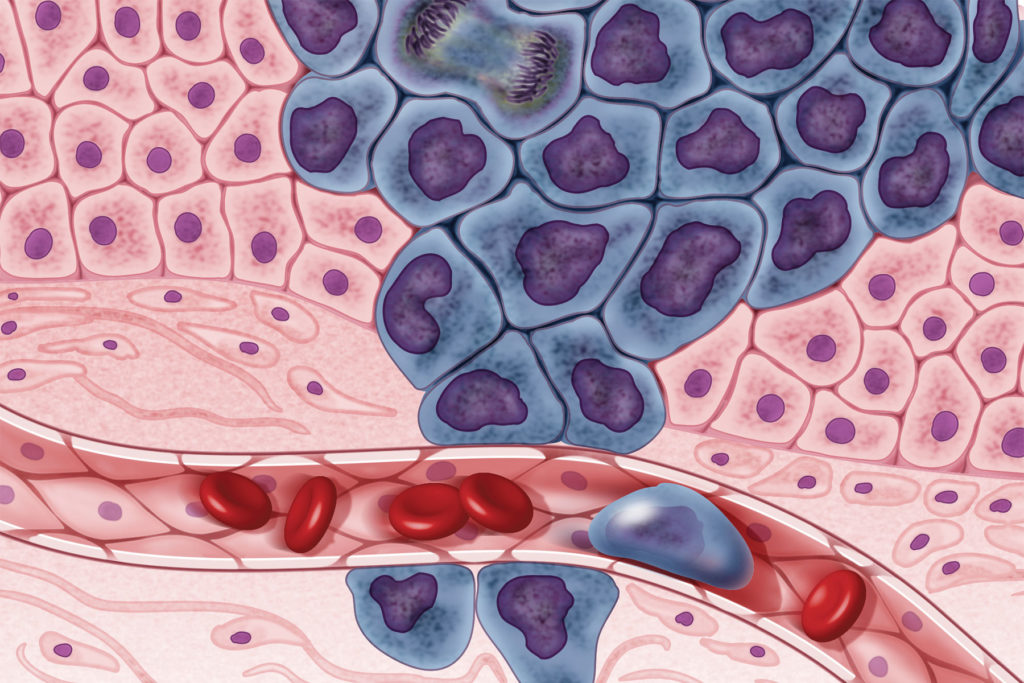
Circulating tumor cells, also known as liquid biopsies, are emerging as an increasingly valuable application for early cancer detection, companion diagnostics, and therapeutic decision-making. As opposed to an invasive tissue biopsy, a liquid biopsy collects a blood sample and examines either cell-free DNA or very rare malignant cells shed by a tumor. However, a blood sample contains very few cancerous cells among many, many blood cells.
“One has to filter out cells that are not of interest and enrich the sample for cancer cells,” says Schwebel. Many cell enrichment strategies are available, and they can isolate a few cancer cells from millions of blood cells. “Any marker,” Schwebel continues, “can then be applied to that sample to analyze the malignant cells, but the challenge becomes deciding which biomarkers to use.”
Selecting the right biomarkers or biomarker combinations can facilitate analysis and even provide the link that has been missing between cell enrichment technologies and microscopy, two fields that have seen extensive expansion over recent years. “This is a decision determined by many questions, including how specific or sensitive the tests are, or whether they can be used for screening,” says Schwebel. “In the case of the more expensive biomarkers used in large populations, it also becomes a question of healthcare economics.”
Stratifying Alzheimer’s disease
“It became apparent several decades ago that dementia is a collection of diseases that includes Alzheimer’s disease, vascular dementia, and frontotemporal dementia,” says Jermaine Ross, PhD, head of neuroscience at Immuneering. “Today, the community is embracing the fact that even Alzheimer’s disease may be a syndrome.”
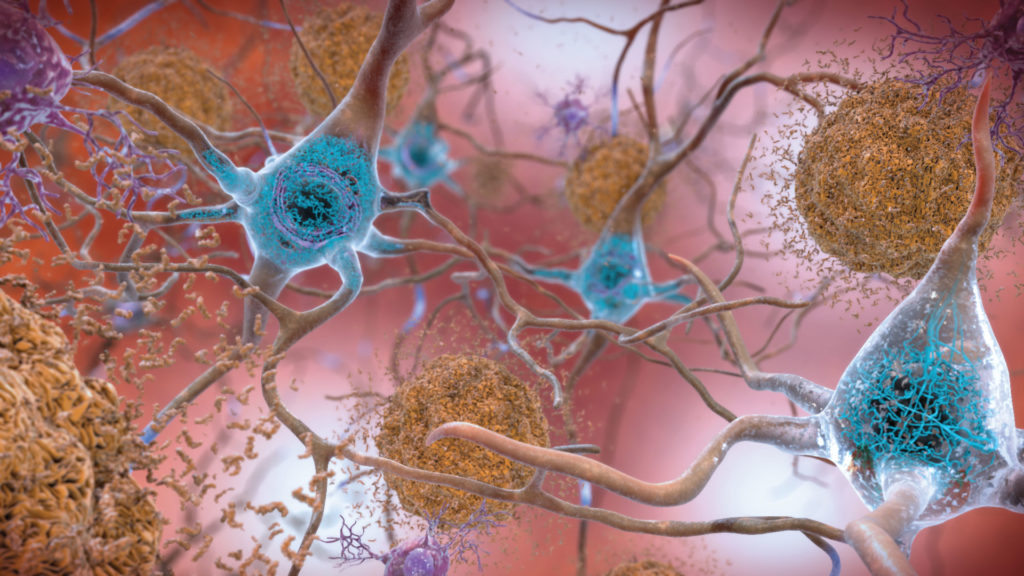
The belief that Alzheimer’s disease may be a constellation of different conditions, rather than a single disease, is motivated by the observation that many parameters, including age of onset, clinical presentation, and disease progression, vary markedly within patient cohorts and can be unique to some types of patients as compared to others. “We wanted to rethink how we define Alzheimer’s disease,” Ross relates, “[and approach it] less on a clinical level and more at a molecular level, to embrace a lot of the data that we collected over several years.”
One of the research efforts at Immuneering is focusing on integrating gene expression data with neuropathological markers to stratify Alzheimer’s disease patient subgroups. This effort, the company hopes, may benefit these subgroups by enabling the identification of therapeutic targets and the development of small-molecule drugs. Embracing the biomarker strategy is critical to the identification of patients who respond to a specific therapy.
“This approach has been used in cancer biology for years to identify individuals who respond differently to a specific medication,” notes Ross. “We wanted to adapt the same strategy to the neuroscience field.”
In the patient data collected, Immuneering scientists found distinct disease signatures at the gene expression level. This strategy may lead to candidates for biomarkers that not only identify individuals more susceptible to developing the disease, but also define patient subgroups more responsive to a particular therapy.
“We have pretty robust biomarkers when it comes to imaging, but some techniques are still cost-prohibitive,” Ross admits. “We want to bring down those costs to make screening much more scalable.”
Proteomics unveils new biomarkers
“As a proteomics technology company, we are developing and inventing new proteomics tools that aim to quantitate proteins and protein modifications better, deeper, and in more samples,” says Oliver Rinner, PhD, founder and CEO of Biognosys. Using mass spectrometry–based proteomics, the Biognosys platform offers two quantitative solutions: discovery proteomics, which provides a label-free workflow to quantitate up to 10,000 proteins per sample, and targeted proteomics, which can quantitate up to 500 predefined proteins in a high-throughput manner from thousands of samples.
Because mass spectrometry does not amplify the signal, it has been historically limited by the quantity of low-abundance proteins that are present in biological fluids such as plasma. “Over 90% of the protein mass is covered by the top 20 proteins,” Rinner notes, “This causes a sensitivity challenge because the more abundant proteins cover the less abundant ones.”
This is one of the reasons why the use of mass spectrometry on biological fluids has been somewhat disappointing in the past. “The technique provided information about the top 200 proteins, and these were often not the most interesting ones,” Rinner adds.
Biognosys scientists have developed a method to remove these high-abundant proteins. “This enables us to see much deeper,” asserts Rinner. “[We can] assay over 1,500 proteins, among them highly interesting proteins that are, for instance, shed from cancer cells.” In tissues, where the dynamic range is not a limiting factor, the technique allows thousands of proteins to be quantified directly.
A major advantage of performing proteomics using mass spectrometry is that by being label-free, it is not affected by many challenges that accompany the development of specific reagents, such as antibodies. Moreover, the possibility of using statistical analyses and applying accurate quantification makes the technique very attractive to investigators interested in quickly analyzing proteins or protein modifications.
“It is also important to look beyond protein quantities and beyond modifications, and to understand how proteins interact, because this can provide a higher-level biomarker,” explains Rinner. In a recent study, Rinner and colleagues developed LiP-Quant, a liquid chromatography–coupled tandem mass spectrometry approach that uses limited proteolysis to detect differential proteolytic patterns generated by the interaction of small molecules with eukaryotic proteomes. This work is part of larger efforts at Biognosys to develop machine-based learning applications that can interrogate proteome-wide the induced structural changes in proteins.
“Proteins change their structure when they bind other proteins or compounds,” notes Rinner, “and one can use mass spectrometry to study these structural changes, which may serve as valuable biomarkers or point to biological pathway activity.”
Biomarkers that interrogate genetic signatures
“Our newest program, RAIN-32, is a potent and selective small-molecule MDM2 inhibitor that we intend to use in genetically predisposed populations” says Avanish Vellanki, CEO and co-founder of Rain Therapeutics, a company that develops targeted, biomarker-driven therapies for patients with cancer. In its biomarker work, the company applies two strategies.
The first strategy involves pursuing cancers that harbor specific genetic modifications known to respond to a therapeutic. For example, cancer types that are anticipated to be most responsive to RAIN-32 are the ones with MDM2 (mouse double minute 2) gene amplifications, such as the well-differentiated/de-differentiated subtype of liposarcoma.
The second strategy “involves taking what we learn from that first approach and trying to understand cancers that are MDM2-dependent using genetic signatures,” says Robert Doebele, MD, PhD, chief scientific officer and co-founder of Rain Therapeutics. Two genetic signatures that Rain Therapeutics scientists are focusing on are MDM2 gene amplification and wild-type p53 status, which tend to be exclusive events in malignancies.
A study that embraces this second strategy proposes to enroll patients with high-level MDM2 amplification and wild-type p53 status, regardless of tumor histology. “This is based on the observation that very diverse cancer types can often be more similar with respect to their biology than to some of their histologic neighbors,” explains Doebele. For example, the genetic signatures of breast and colon cancer may sometimes be more similar than those of two breast cancer samples.
“We have come a long way with comprehensive genomic testing, and we still have a long way to go,” concludes Doebele. “We have only scratched the surface in terms of identifying patient populations based on genetic signatures.”