Say what you will about magnetotactic bacteria, they’re never disoriented. They contain their own compass needles, which are built of linked magnetosomes, nanosized magnetic particles. These magnetosomes may soon point in new directions, thanks to microbiologists at the University of Bayreuth.
These scientists, led by Dirk Schüler, PhD, have developed a modular system for the genetic reprogramming of magnetotactic bacteria. The scientists say that their system can compel the bacteria to produce magnetosomes with desirable properties. Magnetosomes, which are naturally biocompatible, could be modified for use in drug delivery systems, magnetosome‐based immunoassays, and in imaging applications.
The scientist described their work in the journal Small, in an article titled, “A Versatile Toolkit for Controllable and Highly Selective Multifunctionalization of Bacterial Magnetic Nanoparticles.” The article described how Schüler and colleagues used synthetic biology to turn the magnetosome surface into a platform for the “specific versatile display of functional moieties.”
“In this study,” the authors wrote, “a versatile toolkit is developed for the multifunctionalization of magnetic nanoparticles in the magnetotactic bacterium Magnetospirillum gryphiswaldense, and the use of several abundant magnetosome membrane proteins as anchors for functional moieties is explored. High‐level magnetosome display of cargo proteins enables the generation of engineered nanoparticles with several genetically encoded functionalities, including a core–shell structure, magnetization, two different catalytic activities, fluorescence, and the presence of a versatile connector that allows the incorporation into a hydrogel‐based matrix by specific coupling reactions.”
Magnetic bacteria of the species M. gryphiswaldense align their swimming behavior along the Earth’s magnetic field. Within the cells, magnetic nanoparticles, the magnetosomes, are arranged in a chain-like manner, thereby forming an intracellular compass needle. Each magnetosome consists of a magnetic iron oxide core surrounded by a membrane. In addition to lipids, this membrane also contains a variety of different proteins.
Schüler and colleagues report that they have now succeeded in the coupling of biochemically active functional groups, which originate from various foreign organisms, to these proteins. The method used here starts at the stage of the genes that are responsible for the biosynthesis of the membrane proteins. These bacterial genes are fused to foreign genes from other organisms that control the production of the respective functional proteins. As soon as the genes are reintegrated into the genome, the reprogrammed bacteria produce magnetosomes that display these foreign proteins permanently installed on the particle surface.
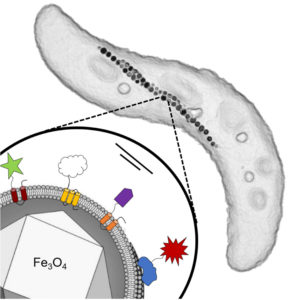
In the study, four different functional groups (that is, foreign proteins) were coupled to the membrane proteins. These include the enzyme glucose oxidase from a mold fungus, which is already used biotechnologically (for example, as a “sugar sensor” in diabetes diseases). In addition, a green fluorescent protein from a jellyfish and a dye-producing enzyme from the bacterium Escherichia coli, whose activity can be easily measured, were installed on the surface of the magnetosomes. The fourth functional group is an antibody fragment from a lama (Alpaca) that was used as a versatile connector. Thus, all these properties including the superb magnetization of the magnetosomes are genetically encoded in the bacteria.
“Using this genetic strategy, we reprogrammed the bacteria to produce magnetosomes that glow green when irradiated with UV light and at the same time display novel biocatalytic functions,” said Schüler. “Various biochemical functions can be precisely installed on their surfaces. Thereby, magnetosomes from living bacteria are transformed into multifunctional nanoparticles with fascinating functions and properties. Moreover, the particles remain fully functional when they are isolated from the bacteria—which can be easily performed by taking advantage of their inherent magnetic properties.”
Functionalization of the magnetosomes by no means is limited to the functional groups that were installed on the particle surface by the Bayreuth microbiologists. Instead, these proteins can easily be replaced by other functions, thus providing a highly versatile platform. Genetic reprogramming, therefore, opens up a broad spectrum to design the magnetosome surface. It provides the basis for a “genetic toolkit” that allows the production of tailored magnetic nanoparticles, combining different useful functions and properties. Each of these particles is between three and five nanometers in size.
“Our genetic engineering approach is highly selective and precise, compared to, for instance, chemical coupling techniques which are not as efficient and lack this high degree of control,” asserted Frank Mickoleit, PhD, the first author of the study.
“Previous studies show that the magnetic nanoparticles are likely not harmful to cell cultures,” he continued. “Good biocompatibility is an important prerequisite for the future application of the particles in biomedicine, for instance as contrast agents in magnetic imaging techniques or as magnetic sensors in diagnostics. In the future, for example, similar particles might help to detect and destroy tumor cells. Bioreactor systems are another field of application. Magnetic nanoparticles equipped with tiny catalysts would be highly suitable for this purpose and enable complex biochemical processes.”
“There is an enormous application potential for nanoparticles that display different functional groups on the surface, particularly in the fields biotechnology and biomedicine,” added co-author Clarissa Lanzloth, who participated in the study while she completed her master’s thesis at Bayreuth. “The magnetic bacteria now may serve as a platform for a versatile nano-toolkit, inspiring scientific creativity in the field of synthetic biology. It will initiate further interesting research approaches.”